
- •Pineal Parenchymal Tumors
- •Germ Cell Tumors
- •Selected References
- •Medulloblastoma
- •Selected References
- •Anatomy of the Cranial Meninges
- •Meningomas
- •Primary Melanocytic Lesions
- •Other Related Neoplasms
- •Selected References
- •Cranial Nerve Anatomy
- •Schwannomas
- •Neurofibromas
- •Selected References
- •Histiocytic Tumors
- •Selected References
- •Sellar Region Anatomy
- •Normal Imaging Variants
- •Congenital Lesions
- •Neoplasms
- •Miscellaneous Lesions
- •Selected References
- •Intracranial Pseudotumors
- •Selected References
- •Metastatic Lesions
- •Paraneoplastic Syndromes
- •Selected References
- •Scalp Cysts
- •Extraaxial Cysts
- •Parenchymal Cysts
- •Intraventricular Cysts
- •Selected References
- •Anatomy and Physiology of the Basal Ganglia and Thalami
- •Selected References
- •Alcohol and Related Disorders
- •Opioids and Derivatives
- •Inhaled Gases and Toxins
- •Selected References
- •Selected References
- •Hypertensive Encephalopathies
- •Glucose Disorders
- •Thyroid Disorders
- •Seizures and Related Disorders
- •Miscellaneous Disorders
- •Selected References
- •The Normal Aging Brain
- •Dementias
- •Degenerative Disorders
- •Selected References
- •Normal Variants
- •Hydrocephalus
- •CSF Leaks and Sequelae
- •Selected References
- •Cerebral Hemisphere Formation
- •Imaging Approach to Brain Malformations
- •Posterior Fossa Anatomy
- •Chiari Malformations
- •Hindbrain Malformations
- •Selected References
- •Commissural Anomalies
- •Malformations Secondary to Abnormal Postmigrational Development
- •Selected References
- •Anencephaly
- •Holoprosencephaly
- •Holoprosencephaly Variants
- •Related Midline Disorders
- •Holoprosencephaly Mimics
- •Selected References
- •Selected References
- •Selected References
- •Cephaloceles
- •Craniosynostoses
- •Meningeal Anomalies
- •Selected References
- •Index

Dementias and Brain Degenerations
Selected References
1117
Ikram MA et al: Genetics of vascular dementia - review from the ICVD working group. BMC Med. 15(1):48, 2017
The Normal Aging Brain
Danka Mohammed CP et al: MicroRNAs in brain aging. Mech Ageing Dev. ePub, 2017
Dekeyzer S et al: "Unforgettable" - a pictorial essay on anatomy and pathology of the hippocampus. Insights Imaging. 8(2):199-212, 2017
Di Benedetto S et al: Contribution of neuroinflammation and immunity to brain aging and the mitigating effects of physical and cognitive interventions. Neurosci Biobehav Rev. 75:114-128, 2017
Hullinger R et al: Molecular and cellular aspects of age-related cognitive decline and Alzheimer's disease. Behav Brain Res. 322(Pt B):191-205, 2017
Lardenoije R et al: The epigenetics of aging and neurodegeneration. Prog Neurobiol. 131:21-64, 2015
Imaging the Normal Aging Brain
Quinn TJ et al: Diagnosis in vascular dementia, applying 'Cochrane diagnosis rules' to 'dementia diagnostic tools'. Clin Sci (Lond). 131(8):729-732, 2017
Roseborough A et al: Associations between amyloid β and white matter hyperintensities: A systematic review. Alzheimers Dement. ePub, 2017
Santos CY et al: Pathophysiologic relationship between Alzheimer's disease, cerebrovascular disease, and cardiovascular risk: a review and synthesis. Alzheimers Dement (Amst). 7:69-87, 2017
Smith EE: Clinical presentations and epidemiology of vascular dementia. Clin Sci (Lond). 131(11):1059-1068, 2017
Søndergaard CB et al: Hereditary cerebral small vessel disease and stroke. Clin Neurol Neurosurg. 155:45-57, 2017
Frontotemporal Lobar Degeneration
Spiegel AM et al: Epigenetic contributions to cognitive aging: disentangling mindspan and lifespan. Learn Mem. 21(10):569-74, 2014
Dementias
Alzheimer Disease
Buckley RF et al: Functional network integrity presages cognitive decline in preclinical Alzheimer disease. Neurology. ePub, 2017
Dallaire-Théroux C et al: Radiological-pathological correlation in Alzheimer's disease: systematic review of antemortem magnetic resonance imaging findings. J Alzheimers Dis. 57(2):575-601, 2017
Dickie DA et al: Whole brain magnetic resonance image atlases: a systematic review of existing atlases and caveats for use in population imaging. Front Neuroinform. 11:1, 2017
Donohue MC et al: Association between elevated brain amyloid and subsequent cognitive decline among cognitively normal persons. JAMA. 317(22):2305-2316, 2017
Masdeu JC: Future directions in imaging neurodegeneration. Curr Neurol Neurosci Rep. 17(1):9, 2017
McKiernan EF et al: 7T MRI for neurodegenerative dementias in vivo: a systematic review of the literature. J Neurol Neurosurg Psychiatry. ePub, 2017
Pagani MM et al: Progressive disgregation of brain networking from normal aging to Alzhimer's disease. Independent component analysis on FDG-PET data. J Nucl Med. ePub, 2017
Sørensen L et al: Differential diagnosis of mild cognitive impairment and Alzheimer's disease using structural MRI cortical thickness, hippocampal shape, hippocampal texture, and volumetry. Neuroimage Clin. 13:470-482, 2016
Lardenoije R et al: The epigenetics of aging and neurodegeneration. Prog Neurobiol. 131:21-64, 2015
Tabatabaei-Jafari H et al: Cerebral atrophy in mild cognitive impairment: a systematic review with meta-analysis. Alzheimers Dement (Amst). 1(4):487-504, 2015
Vascular Dementia
Di Donato I et al: Cerebral autosomal dominant arteriopathy with subcortical infarcts and leukoencephalopathy (CADASIL) as a model of small vessel disease: update on clinical, diagnostic, and management aspects. BMC Med. 15(1):41, 2017
Mann DM et al: Frontotemporal lobar degeneration: pathogenesis, pathology and pathways to phenotype. Brain Pathol. ePub, 2017
Olney NT et al: Frontotemporal dementia. Neurol Clin. 35(2):339374, 2017
Prpar Mihevc S et al: Nuclear trafficking in amyotrophic lateral sclerosis and frontotemporal lobar degeneration. Brain. 140(Pt 1):13-26, 2017
Li YQ et al: Frontotemporal lobar degeneration: mechanisms and therapeutic strategies. Mol Neurobiol. 53(9):6091-6105, 2016
Lewy Body Dementias
Agosta F et al: Advanced magnetic resonance imaging of neurodegenerative diseases. Neurol Sci. 38(1):41-51, 2017
Galasko D: Lewy body disorders. Neurol Clin. 35(2):325-338, 2017
McAleese KE et al: TDP-43 pathology in Alzheimer's disease, dementia with Lewy bodies and ageing. Brain Pathol. 27(4):472479, 2017
Nag S et al: TDP-43 pathology and memory impairment in elders without pathologic diagnoses of AD or FTLD. Neurology. 88(7):653-660, 2017
Gomperts SN: Lewy body dementias: dementia with Lewy bodies and Parkinson disease dementia. Continuum (Minneap Minn). 22(2 Dementia):435-63, 2016
Hogan DB et al: The prevalence and incidence of dementia with Lewy bodies: a systematic review. Can J Neurol Sci. 43 Suppl 1:S8395, 2016
Ingelsson M: Alpha-synuclein oligomers-neurotoxic molecules in Parkinson's disease and other Lewy body disorders. Front Neurosci. 10:408, 2016
Walker Z et al: Lewy body dementias. Lancet. 386(10004):1683-97, 2015
Miscellaneous Dementias
Crutch SJ et al: Consensus classification of posterior cortical atrophy. Alzheimers Dement. ePub, 2017
Gaudino S et al: Neuroradiology of human prion diseases, diagnosis and differential diagnosis. Radiol Med. 122(5):369-385, 2017
Iwasaki Y: Creutzfeldt-Jakob disease. Neuropathology. 37(2):174188, 2017

Toxic, Metabolic, Degenerative, and CSF Disorders
1118
Mead S et al: CJD mimics and chameleons. Pract Neurol. 17(2):113121, 2017
Degenerative Disorders
Pujol S et al: In vivo exploration of the connectivity between the subthalamic nucleus and the globus pallidus in the human brain using multi-fiber tractography. Front Neuroanat. 10:119, 2017
Zwirner J et al: Subthalamic nucleus volumes are highly consistent but decrease age-dependently-a combined magnetic resonance imaging and stereology approach in humans. Hum Brain Mapp. 38(2):909-922, 2017
Plantinga BR et al: Ultra-high field MRI post mortem structural connectivity of the human subthalamic nucleus, substantia nigra, and globus pallidus. Front Neuroanat. 10:66, 2016
Parkinson Disease
Braak H et al: Neuropathological staging of brain pathology in sporadic Parkinson's disease: separating the wheat from the chaff. J Parkinsons Dis. 7(s1):S73-S87, 2017
Frey KA: Molecular imaging of extrapyramidal movement disorders. Semin Nucl Med. 47(1):18-30, 2017
Graebner AK et al: Clinical impact of 123I-Ioflupane SPECT (DaTscan) in a movement disorder center. Neurodegener Dis. 17(1):38-43, 2017
Lill CM: Genetics of Parkinson's disease. Mol Cell Probes. 30(6):386396, 2016
Sako W et al: Imaging-based differential diagnosis between multiple system atrophy and Parkinson's disease. J Neurol Sci. 368:104-8, 2016
Corticobasal Degeneration
Yokoyama JS et al: Shared genetic risk between corticobasal degeneration, progressive supranuclear palsy, and frontotemporal dementia. Acta Neuropathol. 133(5):825-837, 2017
Amyotrophic Lateral Sclerosis
Hardiman O et al: The changing picture of amyotrophic lateral sclerosis: lessons from European registers. J Neurol Neurosurg Psychiatry. ePub, 2017
van Es MA et al: Amyotrophic lateral sclerosis. Lancet. ePub, 2017
Vajda A et al: Genetic testing in ALS: a survey of current practices. Neurology. 88(10):991-999, 2017
Chakraborty S et al: The "motor band sign:" susceptibility-weighted imaging in amyotrophic lateral sclerosis. Can J Neurol Sci. 42(4):260-3, 2015
Wallerian Degeneration
Bekiesinska-Figatowska M et al: Diffusion restriction in the corticospinal tracts and the corpus callosum in neonates after cerebral insult. Brain Dev. 39(3):203-210, 2017
Chen YJ et al: Wallerian degeneration beyond the corticospinal tracts: conventional and advanced MRI findings. J Neuroimaging. 27(3):272-280, 2017
Singh S et al: Relationship of acute axonal damage, Wallerian degeneration, and clinical disability in multiple sclerosis. J Neuroinflammation. 14(1):57, 2017
Tricaud N et al: Wallerian demyelination: chronicle of a cellular cataclysm. Cell Mol Life Sci. ePub, 2017
Booth TC et al: The role of functional dopamine-transporter SPECT imaging in parkinsonian syndromes, part 1. AJNR Am J Neuroradiol. 36(2):229-35, 2015
Multiple System Atrophy
Chen B et al: Usefulness of diffusion-tensor MRI in the diagnosis of Parkinson variant of multiple system atrophy and Parkinson's disease: a valuable tool to differentiate between them? Clin Radiol. 72(7):610.e9-610.e15, 2017
Wang N et al: Using 'swallow-tail' sign and putaminal hypointensity as biomarkers to distinguish multiple system atrophy from idiopathic Parkinson's disease: a susceptibility-weighted imaging study. Eur Radiol. 27(8):3174-3180, 2017
Progressive Supranuclear Palsy
Hall B et al: in vivo tau PET imaging in dementia: pathophysiology, radiotracer quantification, and a systematic review of clinical findings. Ageing Res Rev. 36:50-63, 2017
Lee Y et al: Volumetric analysis of the cerebellum in patients with progressive supranuclear palsy. Eur J Neurol. 24(1):212-218, 2017
Yokoyama JS et al: Shared genetic risk between corticobasal degeneration, progressive supranuclear palsy, and frontotemporal dementia. Acta Neuropathol. 133(5):825-837, 2017
Tipton PW et al: Cerebral peduncle angle: unreliable in differentiating progressive supranuclear palsy from other neurodegenerative diseases. Parkinsonism Relat Disord. 32:31-35, 2016
Hypertrophic Olivary Degeneration
Smets G et al: The dentato-rubro-olivary pathway revisited: new MR imaging observations regarding hypertrophic olivary degeneration. Clin Anat. 30(4):543-549, 2017
Avula S et al: Post-operative pediatric cerebellar mutism syndrome and its association with hypertrophic olivary degeneration. Quant Imaging Med Surg. 6(5):535-544, 2016
Spinocerebellar Ataxias
Beaudin M et al: Systematic review of autosomal recessive ataxias and proposal for a classification. Cerebellum Ataxias. 4:3, 2017
Cerebral Hemiatrophy (Dyke-Davidoff-Masson)
Gökçe E et al: Radiological imaging findings of Dyke-Davidoff- Masson syndrome. Acta Neurol Belg. ePub, 2017
Thakkar PA et al: Dyke-Davidoff-Masson syndrome: a rare cause of cerebral hemiatrophy in children. J Pediatr Neurosci. 11(3):252254, 2016
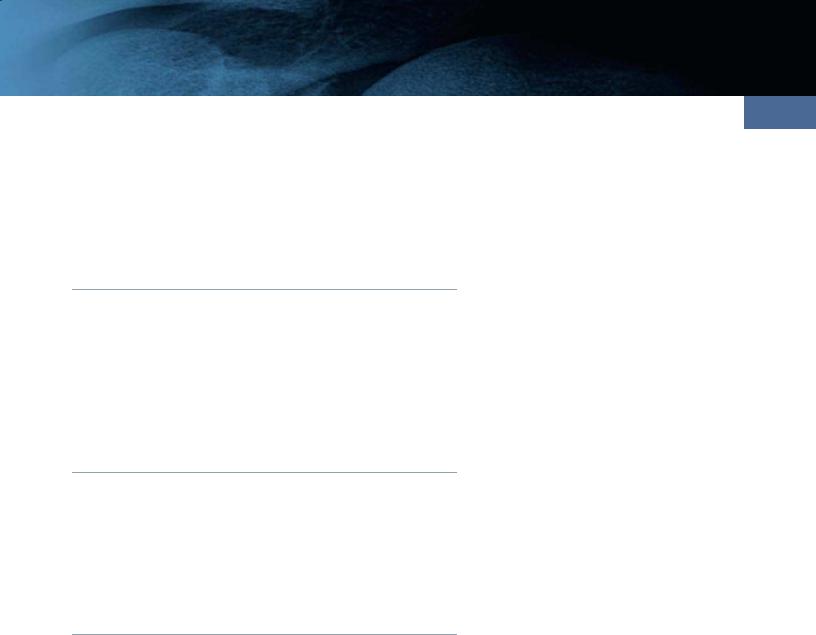
Chapter 34
1119
Hydrocephalus and CSF Disorders
The brain CSF spaces include the ventricular system—a series of interconnected, CSF-filled cavities—and the subarachnoid space. Understanding the normal anatomy of these CSF spaces and their variants is a prerequisite to deciphering their pathology. We therefore begin this chapter with a brief discussion of the normal development of the ventricles and CSF spaces, then delineate their normal gross and imaging anatomy.
We next describe normal variants, which should not be mistaken for disease, then turn our attention to hydrocephalus and the manifestations of elevated CSF pressure, including idiopathic intracranial hypertension ("pseudotumor cerebri"). We close the chapter with a discussion of CSF leaks and intracranial hypotension.
Normal Development of the
Ventricles and Cisterns
Ventricles
The embryonic ventricular system is a series of interconnected fluid-filled chambers that arise as expansions from the central cavity of the embryonic neural tube. As the developing brain bends and expands, it forms forebrain, midbrain, and hindbrain vesicles. The forebrain cavity divides into two lateral ventricles, which develop as outpouchings from the rostral third ventricle and are connected to it by the interventricular foramen (foramen of Monro)
(34-1).
The cerebral aqueduct develops from the midbrain vesicle. The fourth ventricle develops from the hindbrain cavity and merges proximally with the aqueduct and caudally with the central canal of the spinal cord. In the coronal plane, the developing lateral and third ventricles form a central H- shaped monoventricle that continues inferiorly into the aqueduct and then connects to the fourth ventricle.
At the eleventh or twelfth gestational week, the inferomedial aspect of the fourth ventricular roof thins and opens, creating the foramen of Magendie. The foramina of Luschka open shortly thereafter, establishing communication between the developing ventricular system and subarachnoid space.
Normal Development of the |
|
Ventricles and Cisterns |
1119 |
Ventricles |
1119 |
Choroid Plexus |
1120 |
Subarachnoid Spaces |
1120 |
Normal Anatomy of the |
|
Ventricles and Cisterns |
1120 |
Ventricular System |
1120 |
Choroid Plexus, CSF, and Brain |
1121 |
Interstitial Fluid |
|
Subarachnoid Spaces/Cisterns |
1124 |
Normal Variants |
1124 |
Age-Related Changes |
1124 |
Asymmetric Lateral Ventricles |
1124 |
Cavum Septi Pellucidi and |
1124 |
Vergae |
|
Cavum Velum Interpositum |
1126 |
Enlarged Subarachnoid Spaces |
1127 |
CSF Flow Artifacts |
1128 |
Hydrocephalus |
1129 |
Intraventricular Obstructive |
1130 |
Hydrocephalus |
|
Extraventricular Obstructive |
1134 |
Hydrocephalus |
|
Overproduction Hydrocephalus |
1136 |
Normal Pressure Hydrocephalus |
1137 |
Syndrome of Inappropriately |
|
Low-Pressure Acute |
1141 |
Hydrocephalus |
|
Arrested Hydrocephalus |
1142 |
Idiopathic Intracranial |
1142 |
Hypertension |
|
CSF Shunts and Complications |
1144 |
CSF Leaks and Sequelae |
1148 |
CSF Leaks |
1148 |
Intracranial Hypotension |
1149 |
|
|
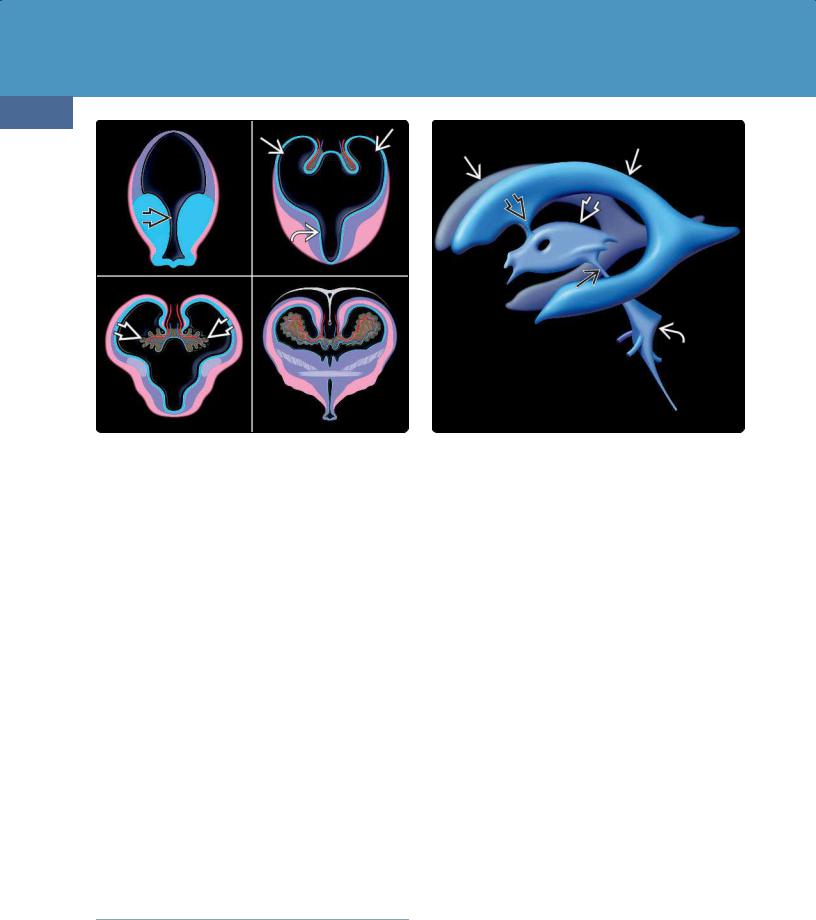
Toxic, Metabolic, Degenerative, and CSF Disorders
1120
(34-1) Embryology of forebrain, ventricles, choroid plexus shows central cavity of neural tube develops outpouchings from rostral 3rd ventricle , forming H-shaped monoventricle.
Choroid plexus develops along choroid fissure.
Choroid Plexus
The embryonic choroid plexus forms where infolded meningeal mesenchyme—the tela choroidea—contacts the ependymal lining of the ventricles. The invagination occurs along the entire choroidal fissure, a narrow cleft that lies in the medial lateral ventricle between the fornix and the thalamus. Initially, the fetal choroid plexus is large relative to the size of the lateral ventricles, occupying nearly threequarters of the ventricular lumen. As the brain and ventricular system grow, the choroid plexus gradually diminishes in relative volume.
Subarachnoid Spaces
The leptomeninges are derived from a gelatinous layer of paraxial mesoderm—the primary meninx or "meninx primitiva"—that envelops the neural tube. At gestational day 32, the innermost zone of the primary meninx systematically degenerates, forming irregular spaces on the ventral aspect of the rhombencephalon. These spaces then extend caudally and dorsally, eventually coalescing to form the fluid-filled leptomeninges.
Normal Anatomy of the
Ventricles and Cisterns
Ventricular System
(34-2) Graphic depicts the paired lateral ventricles , foramen of Monro , third ventricle , aqueduct , fourth ventricle with its three-outlet foramina, and inferiorly directed obex.
ventricle via the Y-shaped foramen of Monro. The third ventricle communicates with the fourth ventricle via the cerebral aqueduct (of Sylvius). In turn, the fourth ventricle communicates with the subarachnoid space.
Lateral Ventricles
Each lateral ventricle is a C-shaped structure with a body, atrium, and three projections ("horns"). We consider each part of the lateral ventricle from front to back.
The body of the lateral ventricle passes posteriorly under the corpus callosum. Its floor is formed by the dorsal thalamus, and its medial wall is bordered by the fornix. Laterally, it curves around the body and tail of the caudate nucleus.
The atrium contains the choroid plexus glomus and is formed by the convergence of the body with the temporal and occipital horns. The temporal horn extends anteroinferiorly from the atrium and is bordered on its floor and medial wall by the hippocampus. The occipital horn is surrounded entirely by white matter tracts, principally the geniculocalcarine tract and the forceps major of the corpus callosum.
The frontal horn is the most anterior segment of the lateral ventricle. Its roof is formed by the corpus callosum genu, and it is bordered inferolaterally by the head of the caudate nucleus. The septi pellucidi are thin bilayered membranes that extend from the corpus callosum to the foramen of Monro, forming the medial borders of both frontal horns.
Foramen of Monro
The ventricular system is composed of four interconnected ependyma-lined cavities that lie deep within the brain (34-2). The paired lateral ventricles communicate with the third
The foramen of Monro (interventricular foramen) is a Y- shaped structure with two long arms extending toward each lateral ventricle and a short inferior common stem that

Hydrocephalus and CSF Disorders
1121
(34-3) Sagittal graphic depicts subarachnoid spaces with CSF (blue) between arachnoid (purple) and pia (orange). The pia is closely adherent to the brain, while the arachnoid loosely adheres to the dura.
connects with the roof of the third ventricle. The anterior borders of the foramen on Monro are formed by the pillars (bodies) of the fornices. The posterior border is formed by the choroid plexus.
Third Ventricle
The third ventricle is a single, slit-like, midline, vertically oriented cavity that lies between the thalami. Its roof is formed by the tela choroidea, a double layer of invaginated pia. The anterior commissure lies along the anterior border of the third ventricle. The floor of the third ventricle is formed by the optic chiasm, hypothalamus, mammillary bodies, and roof of the midbrain tegmentum.
The third ventricle has two inferiorly located projections, the slightly rounded optic recess and the more pointed infundibular recess. Two small recesses, the suprapineal and pineal recesses, form the posterior border of the third ventricle. A variably sized interthalamic adhesion (the massa intermedia) lies between the lateral walls of the third ventricle.
Cerebral Aqueduct
The cerebral aqueduct is an elongated tubular conduit that lies between the midbrain tegmentum and the quadrigeminal plate. It connects the third ventricle with the fourth ventricle.
Fourth Ventricle
The fourth ventricle—sometimes called the rhomboid fossa—is a diamond-shaped cavity that lies between the dorsal pons and the vermis (34-3) (34-4). The fourth ventricle has five distinct recesses. The fastigium is a prominent
(34-4) Sagittal T2WI shows lateral ventricle , velum interpositum , "pointed" recesses of the anterior third ventricle , and fastigium of the fourth ventricle .
triangular dorsal midline outpouching that points toward the vermis. The posterior superior recesses are paired, slender, CSF-filled pouches that curve over the cerebellar tonsils. The lateral recesses curve anterolaterally from the fourth ventricle, passing under the major cerebellar peduncles into the lower cerebellopontine angle cisterns (34-5).
The fourth ventricle gradually narrows as it courses inferiorly, forming the obex. Near the cervicomedullary junction, the obex becomes continuous with the central canal of the spinal cord. The junction between the obex and central canal is demarcated by a prominent dorsal "bump" formed by the nucleus gracilis.
Choroid Plexus, CSF, and Brain
Interstitial Fluid
The CSF space is a dynamic pressure system with a hydrostatic balance between CSF production and absorption. CSF pressure determines intracranial pressure. With lumbar puncture, the CSF should appear clear with opening pressure ranging from 3-4 mm Hg before 1 year of age to 10-15 mm Hg (100-180 mm H O) in adults.
Anatomy
The choroid plexus (CP) is composed of numerous highly vascular papillary or frond-like excrescences. These papillae consist of a central connective tissue core covered by ependyma-derived secretory epithelium.
CP is present in all parts of the ventricular system except for the cerebral aqueduct and the frontal and occipital horns of the lateral ventricles.
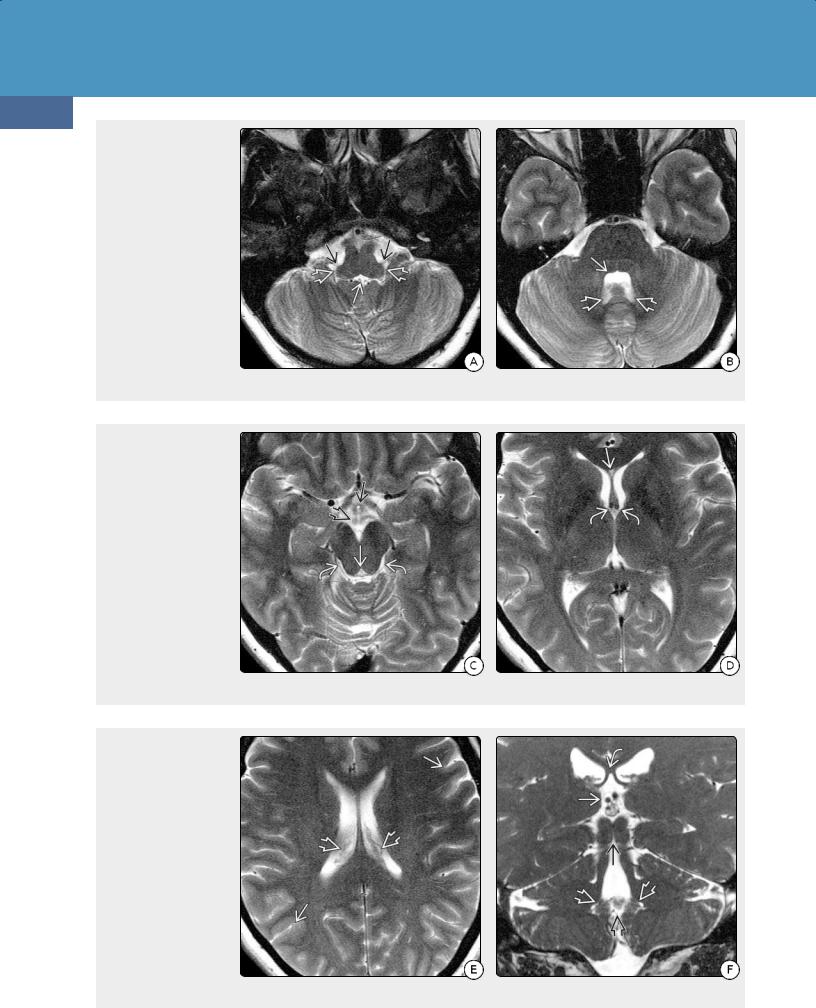
Toxic, Metabolic, Degenerative, and CSF Disorders
1122
(34-5A) These 3.0-T T2 scans demonstrate normal ventricular anatomy. Inferior fourth ventriclecontains small "dots" of choroid plexus. The posterolateral recesses and foramina of Luschkaare indicated. (34-5B) The body of the fourth ventricle resembles a kidney bean on its side. Note indentations caused by the facial colliculi . The posterior superior recesses cap the cerebellar tonsils.
(34-5C) Suprasellar cistern with the hypothalamus and infundibular recess of 3rd ventricle , mammillary bodies are clearly seen. Cerebral aqueduct is small and triangular . Quadrigeminal cisterns contain choroidal arteries, basal vein of Rosenthal, and the trochlear nerves. (34-5D) Frontal horns of the lateral ventricles are separated by the septi pellucidi. A tiny CSP is present . Note foramen of Monro with its two connections .
(34-5E) Choroid plexus, vessels course anteromedially toward the foramen of Monro (seen on lower section). Surface sulci are small but well delineated in this normal scan. (34-5F) Coronal scan shows velum interpositum lying below fornices . The rhomboid 4th ventricle joins the aqueduct above. Midline foramen of Magendie , posterosuperior recessescapping cerebellar tonsils are shown.
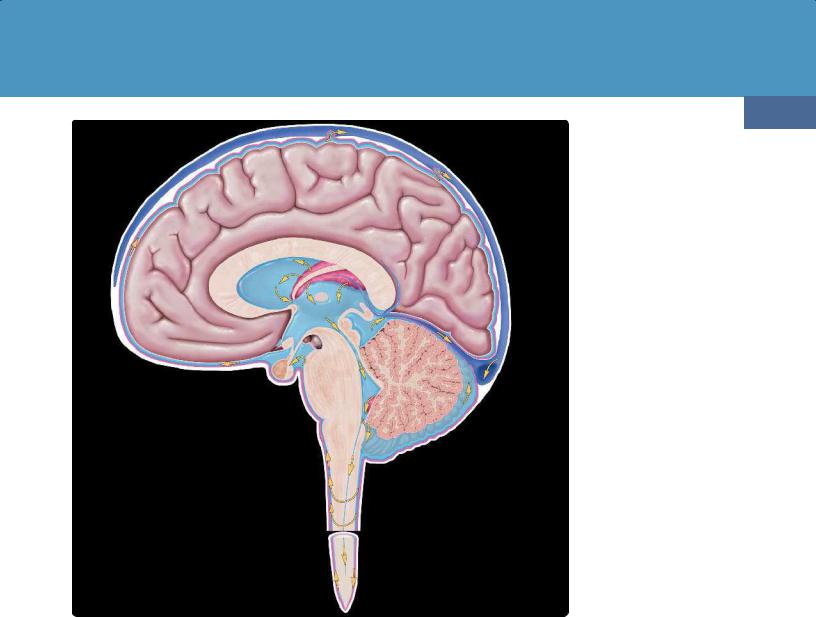
Hydrocephalus and CSF Disorders
The largest mass of CP is the glomus, located in the atrium of the lateral ventricles. The CP extends anterosuperiorly from the glomus along the floor of the lateral ventricle, lying between the fornix and the thalamus. It also extends anteroinferiorly from the glomus into the temporal horn, where it fills the choroidal fissure and lies superomedial to the hippocampus.
The CP of the lateral ventricles passes into and through the foramen of Monro, curving posteriorly along the roof of the third ventricle and terminating near the suprapineal recess. The third ventricle CP is often hypoplastic and inapparent on imaging studies.
The CP of the fourth ventricle extends from the midline, protruding laterally through the foramen of Luschka into the cerebellopontine angle cisterns. These tufts of choroid plexus are located anterior to the flocculus, inferolateral to the CN VII/VIII complex, and posterosuperior to the CN IX, X, and XI complex.
Function
The CP has two major functions: CSF production and maintenance of the blood-CSF barrier. In adult humans, the CP epithelium forms CSF at the rate of about 0.4 mL per minute
1123
(34-6) Sagittal graphic depicts classic model of normal CSF circulation. In this model, CSF is mostly produced by the lateral ventricular choroid plexus and flows in a unidirectional manner from the lateral ventricles through the short Y-shaped foramen of Monro into the third ventricle. From there, CSF passes through the long, narrow cerebral aqueduct into the fourth ventricle. The fourth ventricle has three outlet foramina, the midline foramen of Magendie and the two lateral foramina of Luschka. When CSF exits the fourth ventricle into the cisterna magna, it circulates throughout the subarachnoid cisterns, including those in the spinal canal. CSF is eventually resorbed primarily through the arachnoid granulations into the dural venous sinuses. In newer models of brain CSFinterstitial fluid (ISF) homeostasis, some CSF is probably absorbed into the brain and passes via the paravascular spaces through the cribriform plate into the cervical lymphatics.
or about 500-600 mL every 24 hours. CSF is turned over about four times a day, allowing for the removal of waste products.
The CP is not the only source of CSF. Brain interstitial fluid (ISF) is a significant extrachoroidal source of CSF. Smaller potential sources of CSF production include the ventricular ependyma and brain capillaries.
In adult humans, there are 280 mL of ISF and 140 mL of CSF, of which 30 mL are in the ventricles, 80 mL in the cerebral subarachnoid space (SAS), and 30 mL in the spinal SAS.
The CP maintains the blood-CSF barrier via tight junctions between epithelial cells. The exchange of substances between the brain extracellular fluid and the CSF across the blood-CSF barrier is highly regulated. Specialized subpopulations of CP epithelial cells are responsible for the transfer of plasma proteins from blood to the CSF via the albumin-binding protein SPARC.
CSF Circulation and Homeostasis
Traditional Model of CSF Homeostasis. The longstanding, classic model of CSF homeostasis was based on the circulation theory in which the majority of CSF is produced by the CP, then circulates from the ventricles into the SASs. In this model, CSF