
книги / Структурно-механические свойства эластомерных композитных материалов
..pdf
вания. Оптимальные значения параметров фракций приведены в табл. 1. Выбрана стандартная относительная скорость деформирования –
1,4 10 3 c 1. В табл. 2 приведены начальные данные для расчета энергии разрушения.
b , %
Рис. 4. Расчётная зависимость энергии механического разрушения (W) наполненного диоксидом кремния трёхмерно сшитого эластомера
от разрывной деформации ( b ) при температурах опыта:
– Т =223 К;
– Т=273 К;
– Т=323 К
На рис. 4 показана зависимость энергии разрушения наполненного эластомера от разрывной деформации образца ( b ) при прочих равных
условиях (см. С1 и С2), как и в формулах (3), (4). Видно, что в отличие от огибающих точек разрушения по Смиту [11] и [15] энергия разрушения более полно, в физическом смысле, отражает механическое сопротивление наполненного эластомера, что важно при оценке его эксплуатационной пригодности в конкретных материалах и изделиях.
Указанная зависимость позволяет оценить влияние b на величину W . Так, например, изменение b от 30 до 60 % (в два раза) приво-
дит к относительному возрастанию величины W от 0,32 до 1,05, т.е. в 3,2 раза, что указывает на более медленное уменьшение разрывного на-
131

пряжения, связанного с разрывной деформацией на огибающих точек разрушения. Механизм этого явления требует дальнейших исследований.
Таблица 2. Оптимальные значения параметров фракции диоксида кремния
№ фракции |
Диаметр частиц, мкм |
|
Объёмная |
|
Объемная |
|
|
|
доля пор |
|
доля фракции |
1 |
1 |
|
0,465 |
|
0,079 |
2 |
30 |
|
0,386 |
|
0,237 |
3 |
600 |
|
0,360 |
|
0,684 |
Таблица 3. Начальные данные для расчета энергии |
|||||
|
механического разрушения |
|
|||
|
|
|
|
||
Количество фракций |
|
|
3 |
||
Температуры опыта, К |
|
|
223, 273, 323 |
||
Температура стеклования полимера, К |
|
|
175 |
||
Температура стеклования пластификатора, К |
180 |
||||
Коэффициент объемного расширения полимера |
5·10–4 |
||||
Коэффициент объемного расширения пластификатора |
7·10–4 |
||||
Объемная доля полимера в связующем |
|
|
0,7 |
||
Температура стеклования полимерного связующего, К |
177 |
||||
Объемная доля наполнителя в композите |
0,65 |
||||
Эффективная концентрация поперечных связей в свя- |
1·10–5 |
||||
зующем, моль/см3 |
|
|
|
||
Максимальное объёмное наполнение |
|
|
0,93 |
Важно отметить следующее. Увеличению разрывного удлинения наполненного трёхмерно сшитого эластомера в соответствии с уравнением (1) способствует уменьшение значений основных структурных параметров – ch , r , / m , – а также температуры структурного стеклова-
ния полимерного связующего. При этом снижение разрывного напряжения ( b ) , связанного с b формулой:
b C1(1 C2 b 1)( b b 2 )
132
при принятых ранее обозначениях применительно к уравнению (1), происходит в меньшей степени. Этим, видимо, можно объяснить соответствующее возрастание значения W при росте b . Полученную на теорети-
ческой основе зависимость (5) энергии механического разрушения трёхмерно сшитого наполненного эластомера от основных структурных параметров композита можно рекомендовать для решения прямой и обратной задач при проектировании новых полимерных композитов различного назначения с требуемым комплексом эксплуатационных характеристик [1]. При этом целесообразно пользоваться компьютерными программами, включающими математические методы оптимизации [9, 22, 23]. Естественно, что это приведёт к существенному сокращению сроков разработки и экономии сырья, материалов, например, при создании материала деформационного шва и гидроизоляционного морозостойкого покрытия асфальта с целью многократного повышения эксплуатационного ресурса автомобильных дорог [16, 24].
ВЫВОДЫ
Впервые представлено физико-математическое обоснование численного моделирования процесса механического разрушения эластомера, наполненного твёрдыми частицами, в частности, диоксида кремния полифракционного состава. Дан инженерный пример расчёта диаграмм растяжения эластомерного композита на основе трёхмерно сшитых низкомолекулярных каучуков в зависимости от степени отслоения частиц крупной фракции диоксида кремния.
Впервые в теории наполненных эластомеров выведено уравнение, связывающее его энергию механическую и его основные параметры состава. Предложена обобщённая зависимость разрывной деформации полимерного связующего от эффективной концентрации поперечных химических и межмолекулярных связей, необходимая при инженерном проектировании новых композитов.
СПИСОК ЛИТЕРАТУРЫ
1.Ермилов А. С., Нуруллаев Э. М. // Механика композитных мате-
риалов. 2012.Т. 48. № 3. С. 359–372.
2.Staverman A.J. Heijboer J. // Kunstoffe. 1960. B. 50. PP. 23–30.
133
3.Sato Y., Furukawa J. A. // Rubber Chem. Techn. 1962. Vol. 35. Nо. 3. PP. 857–877.
4.Sato Y., Furkawa J. A. // Rubber Chem. Techn. 1963. Vol. 36. Nо. 4. PP. 1081–1106.
5.Farris R. J. // Trans. Soc. Rheology. 1968. Vol. 12. Nо. 2. PP. 303–314.
6.Farris R. J. // Trans. Soc. Rhelogy. 1968. Vol. 12. Nо. 2. PP. 315–334.
7.Oberth A. E. // Rubber Chem. Techn. 1967. Vol. 40. Nо 5. P. 1337–
1363.
8.Сакович Г. В. // Докл. АН СССР. Серия химическая. 1990. № 10.
С. 2355–2375.
9.Ермилов А. С., Нуруллаев Э. М., Субботина Т. Э, Дурегин К. А. Свидетельство № 2011615640 РФ. Математическое программное обеспечение прогнозирования физико-механических характеристик наполненных эластомеров. / Приоритет от 18.07.2011 г.
10.Smith T. L. // Amer. Soc. Test. Mat. Spec. Publ.1962. N. 325. PP. 60–89.
11.Смит Т. Л. Механические свйства новых материалов. М.: Мир, 1966. 1190 с.
12.Нильсен Л.Е. Механические свойства полимеров и полимерных композиций. М.: Химия, 1978. 310 с.
13.Ермилов А. С., Федосеев А. М. // Журнал прикладной химии. 2004. Т.77. Вып. 7. С. 1218–1220.
14.Ermilov A. S., Nurullaev E. M. // Fracture and Structural Integrity. 2013. N. 24. PP. 69–74.
15.Ермилов А. С., Нуруллаев Э. М. // Журнал прикладной химии. 2012. Т. 85. Вып. 8. С. 1371–1374.
16.Патент № 2473581 РФ. Гидроизоляционное морозостойкое покрытие асфальта автомобильной дороги / Ермилов А. С., Нуруллаев Э. М., Аликин В. Н. Приоритет от 31. 05. 2011 г.
17.Ренней М., Бергер С., Марсден И. Поверхности раздела в полимерных композициях. Том 6. М.: Мир, 1978. С. 140–180.
18.Бронштейн И. Н., Семендяев К. А. Справочник по математике для инженеров и учащихся ВТУЗов. М: Наука. 1986. 544 с.
19.Забродин В. Б., Зыков В. И., Чуй Г. Н. // Высокомолекулярные соединения. 1975. Т. (А) XVII. № 1. С. 163–169.
20.Ван Кревелен Д. В. Свойства и химическое строение полимеров / пер. с англ. под ред. Малкина А. Я. / М.: Химия, 1976. 415 с.
134
21.Мэнсон Д. А., Сперлинг Л. Ч. Полимерные смеси и композиты.
М.: Химия, 1979. 440 с.
22.Ermilov A. S., Nurullev E. M. // Mechanics of Composite Materials. 2013. Vol. 49. No. 3. PP. 455 – 464.
23.Ермилов А. С., Нуруллаев Э. М., Дурегин К. А. Свидетельство
№2012613349 РФ. Программное обеспечение определения и оптимизации плотности упаковки твёрдых дисперсных наполнителей полимерных композиционных материалов (Реология). /Приоритет от 09.04.2012 г.
24.Ермилов А. С., Нуруллаев Э. М. Научные основы создания морозогидроустойчивого покрытия. Физико-химические исследования в области создания морозо-гидроустойчивого покрытия асфальта автомо-
бильных дорог. Gamburg: LAP LAMBERT Academic Publishing GmbH & Co. KG, P. 2012. 183с.
Mechanics of Composite Materials, 2014, Vol. 50, No. 6
ENERGY OF THE MECHANICAL DESTRUCTION OF AN ELASTOMER FILLED WITH SOLID PARTICLES
A. S. Ermilov and E. M. Nurullaev*
Keywords: filled elastomer, fracture energy, multifractional silica, ultimate elongation.
For the first time, a theoretical description of the mechanical destruction of a spatially cross-linked plasticized elastomer filled with solid particles in relation to the basic structural parameters of the composition is presented. The corresponding integral equation is solved using an appropriate stress–strain relation in uniaxial tension. Engineering applications of the theory to waterproof frost-resistant coatings and seams of asphalt motor roads are considered.
INTRODUCTION
Polymer composite materials on the basis of a spatially cross-linked elastomeric matrix filled with solid particles are widely employed in parts and units of various structures of aircraft, shipbuilding, and automotive industries. Rubber automobile tires are among the most successful engineering achievements in
135
this area. Elastomers filled with multifractional silica are used in the building industry, including roof coverings of buildings and floorings in sports structures.
Mechanical characteristics of these materials greatly affect the service life of particular parts or units of structures. In this case, the most important structural parameters are the molecular structure of the polymeric base, the type and degree of its plasticization, the volume degree of effective filling, which depends on the form and fractional composition of disperse filler particles, and on the physical and chemical interaction at the elastomer-filler interface [1].
The structural-mechanical relation makes it possible to solve the direct and inverse problems of optimization in developing new polymer composites with a required complex of operational properties (see, for example, [2]).
In estimating the mechanical characteristics in uniaxial tension, which is the most widespread type of tests, it is reasonable to construct the envelope of destruction points of specimens according to Smith [3-6], which are determined at different temperatures and deformation rates, in the log(σb/T0) – log(αb−1), coordinates, where σb and αb are the rupture stress and the stretch ratio; T and T0 are the temperature of tests and an arbitrary temperature of comparison. Thus, it turns out that the envelope of rupture points of specimens is indirectly related to the area under the tension diagram, i.e., to the energy (work) of mechanical destruction (fracture energy) of a polymer composite. In this connection, the aim of this study is a quantitative estimation of the energy of mechanical destruction of a filled elastomer, which corresponds to the area under the tension diagram of a specimen. For this purpose, we solved an integral equation of certain kind with the use of a known relation between the stress and stretch ratio of a specimen specified in temperature-deformation rate conditions.
THEORY
Let us examine a variant of conservation of continuity of a composite material up to destruction, which is of greatest practical interest for increasing its operational life (the Poisson ratio →05.).
In [1], a physical and mathematical description of the conditional (related to the initial cross section) stress s as a function of stretch ratio a of the specimen of a filled elastomer with account of its basic structural parameters is presented:
136
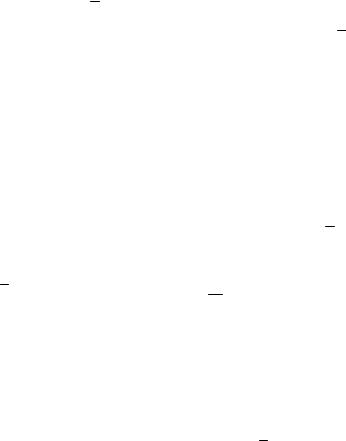
1/3 |
|
|
|
|
|
3 |
T Tg |
2 |
|
1 1 |
|
|
ch r |
RT 1 29exp 0,225 |
10 |
|
|
|
|
||||||
|
|
|
/ m |
2 |
2 , |
|
|
|
(1) |
|||
|
1 |
1,25 |
|
|
|
|
|
|
||||
|
|
|
|
|
|
|
||||||
|
|
|
1 / m |
|
|
|
|
|
|
|
|
where ch / Mc is the concentration of transverse chemical bonds in the
polymeric base of binder; ρ is the density of polymer; Mc is the average inter-
stitial molecular mass of a spatially cross-linked polymer; φr = (φsw – 1) is the volume fraction of polymer in the binder; φsw is the volume fraction of softener in the binder; R is the universal gas constant; T∞ is the equilibrium temperature at which the intermolecular interaction νph in the binder is negligibly low (νph →0); T is the testing temperature of a specimen; Tg is the structural glasstransition temperature of the binder; a is a factor of high-speed displacement;
φ is the volume fraction of filler; φm is the maximun possible (ultimate) volume fraction of filler, which depends on the form and fractional structure of
filler particles. In practice, the structural parameter Mc is found by means of the molecular graph [1]
[Mc ] [( f 32)2 R3 f32 R2 f21 (R1 f12 R2)n f23 R3 ( f32 )2] ,
where R1 and R2 are molecular chains of two rubbers with two end functional groups f1 and f2; R3 is the molecule of a cross-linking agent with three functional groups f3; the combinations of subscripts of f mean the products of chemical reactions of ith and jth antipode end functional groups of bifunctional polymers of sorts R1 and R2 and of the cross-linking threefunctional agent of sort R3. Taking into account the fact that usually R3<< R1
and R2, we may assume that the quantity Mc is proportional to the increment
of the molar fraction of linear polymerization (–R1 – f12 –R2 –)n according to the molecular graph.
The quantity φm can be either calculated [7] or determined by the method of viscometry [8]. The stretch ratio a is connected with e by the known relation α = 1 + ε /100 Under the condition of conservation of continuity of the material (the Poisson ratio →05.), the true stress σver is equal to the product σα; however, in practice, it is more convenient to use the conditional stress for comparison with investigation results for composites which do not retain their continuity up to rupture [1]. Based on Eq. (1), we can write the relation
137
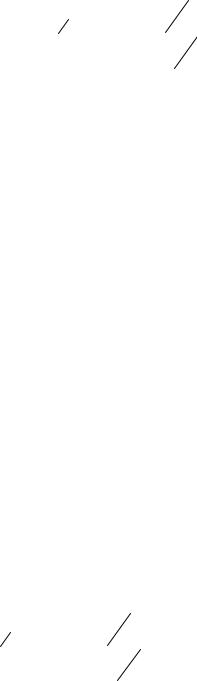
|
|
|
|
b |
|
|
|
|
|
|
b |
|
|
|
|
|
|
|
|
|
|
|
|
2 |
|
|
|
|
|
|
|
W |
|
|
|
|
d |
|
|
|
13 RT |
1 |
1,25 |
|
|
m |
|
|
|
|
|
|
|
||||||
|
|
|
|
|
|
|
|
|
|
|
|
|
|
||||||||||||||||
|
|
ch |
|
|
|
|
|
|
|
|
|
||||||||||||||||||
|
|
|
|
|
|
|
|
|
|
|
r |
|
|
|
|
1 |
|
|
|
|
|
|
|
|
|||||
|
|
|
|
1 |
|
|
|
|
|
|
1 |
|
|
|
|
|
|
|
|
|
|
|
|
|
|
(2) |
|||
|
|
|
|
|
|
|
|
|
|
|
|
|
|
|
|
|
|
|
|
|
|
m |
|
|
|
|
|||
|
3 |
3 b 2 |
|
|
|
|
|
|
|
|
|
|
3 |
|
|
2 |
|
|
1 |
|
3 |
|
|
2 |
1 |
|
|||
|
b |
|
|
|
|
|
|
|
|
|
T |
Tg |
|
|
|
2 b |
3 b |
|
|||||||||||
|
|
2 |
|
|
29exp 0,225 10 |
|
|
|
a |
|
|
2 |
2 |
|
|
||||||||||||||
|
|
b |
|
|
|
|
|
|
|
|
|
|
|
|
|
|
|
|
|
|
|
|
|
b |
|
|
|||
|
|
|
|
|
|
|
|
|
|
|
|
|
|
|
|
|
|
|
|
|
|
|
|
|
|
where W is the energy (work) of mechanical destruction in uniaxial tension, whose dimension is MPa × elongation (mm)3 = 1·103 J. The latter serves as an equivalent of the time of resistance of a polymer composite to the increasing tensile stress.
Assuming that the quantities W and a are variable and designating the combination of the remaining alphabetic symbols and numbers by constants С1 and С2, we have from Eq. (2)
C |
1/3RT |
|
|
/ m |
|
|
|
2 |
; |
|
|
|
|
||||||
1 1,25 |
|
|
|
|
|
|
|
||||||||||||
|
|
|
|
|
|
|
|
|
|
|
|
||||||||
1 |
|
ch r |
|
|
|
|
1 / m |
|
|
|
|
|
|
|
|
|
|||
|
|
|
|
|
|
|
|
|
|
|
|
|
|
|
|
||||
|
|
|
0,225 10 |
3 |
(T Tg ) |
2 |
|
1 |
, |
|
|
|
|||||||
C2 29exp |
|
|
a |
|
|
|
|||||||||||||
wherefrom |
|
|
|
|
|
|
|
|
|
|
|
|
|
|
|
|
|
|
|
|
|
b |
|
|
|
|
|
|
|
|
|
|
|
|
|
|
|
|
|
W C1(1 C2 1)( 2 )d . |
|
|
|
|
(3) |
||||||||||||||
|
|
1 |
|
|
|
|
|
|
|
|
|
|
|
|
|
|
|
|
|
Equation (3) can be solved by using the table of basic integrals [9]. As a |
|||||||||||||||||||
result of integration and algebraic transformations, we have |
|
|
|||||||||||||||||
|
3 |
3 |
b |
2 |
|
|
|
|
2 3 3 |
2 1 |
|
(4) |
|||||||
W C1 |
b |
|
|
C1C2 |
|
b |
|
|
b |
|
|
. |
|||||||
|
2 b |
|
|
|
2 |
|
|
|
|||||||||||
|
|
|
|
|
|
|
2 b |
|
|
|
|
|
|||||||
In view of designations in (2), from here follows the required relation |
between the energy of mechanical destruction of a filled elastomer and its basic structural parameters
|
|
|
|
|
|
|
|
|
|
|
|
|
|
2 |
|
|
|
|
|
|
|
|
|
|
|
|
W |
|
13 RT 1 1,25 |
|
m |
|
|
|
|
|
|
|
|
|
|
|
|||
|
|
|
|
ch |
|
|
|
|
|
|
|
|
|
|
|
|
||||||
|
|
|
|
|
|
|
|
1 |
|
|
|
|
|
|
|
|
|
|
||||
|
|
|
|
|
r |
|
|
|
|
|
|
|
|
|
|
|
|
|
|
|
|
|
|
|
|
|
|
|
|
|
|
|
|
m |
|
|
|
|
|
|
|
(5) |
|||
|
3 |
3 b 2 |
|
|
|
|
3 |
|
|
|
2 |
|
1 |
|
3 |
|
|
2 |
1 |
|
||
|
b |
|
|
10 |
T |
Tg |
|
|
2 b |
3 b |
|
|||||||||||
|
|
2 |
|
29exp 0,22 |
|
|
a |
|
2 |
2 |
|
|
||||||||||
|
|
b |
|
|
|
|
|
|
|
|
|
|
|
|
|
b |
|
|
||||
|
|
|
|
|
|
|
|
|
|
|
|
|
|
|
|
|
|
|
138

The fracture energy W is equal to zero at αb=1, which means that it is normalized.
The limiting values of the stretch ratio αb and strain εb can be estimated by taking into account the rate and magnitude of deformation of an average interlayer of polymeric binder between solid particles of the filler [1]:
af a0 (1 3 / m ) 3 / m ;
bf b0 (1 3 / m ) 3 / m ; |
(6) |
bf b0 (1 3 / m ),
where the indices “f” and “0” denote the filled and free states of the spatially cross-linked polymeric binder.
ULTIMATE ELONGATION AND FRACTURE ENERGY
For engineering applications of Eq. (5) in designing new composites on the basis of spatially cross-linked elastomers, in particular, for waterproof and frost-resistant asphalt coverings of highways [19], it is necessary to know the limiting stretch ratio or the rupture strain of the polymeric binder. As follows
from Eq. (1), the values of b0 or b0 depend on the effective concentration νeff
of transverse chemical bonds, which determine the temperature dependence of mechanical characteristics:
eff |
1/3 |
1/3 |
1 29exp |
|
10 |
3 |
(T Tg ) |
2 |
ch r |
(1 ph ) ch r |
0,225 |
|
. (7) |
where Tg is the structural glass-transition temperature of the polymeric binder, which depends on the physical and chemical properties of its components, including the softener, and on the chemical structure of the molecular network of the polymeric base.
The parameters of the molecular structure, including the average interstitial molecular weight Mc , of spatially cross-linked systems based on low-
molecular polymers with end functional groups were theoretically evaluated in [11]. However, the authors did not take into account the intermolecular interaction which, as well as the mechanical properties, depends on a number of factors [12-14], as was already mentioned. Therefore, in order to determine the
139
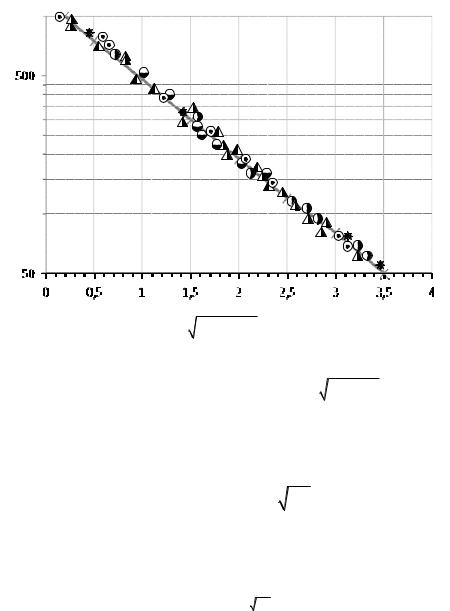
rupture strain of a free polymeric binder as a function of veff in the engineering practice, we generalized the experimental data obtained in [1]. It turned out
that the nonlinear experimental relation b0 (%) = f (νeff) for different polymer-
ic binders, constructed in the logarithmic scale [1], became linear in the coordinates
b0,%
( eff |
10 3 ), |
|
|
Fig. 1. Experimental relation between |
the rupture strain b0 and |
the effective |
|
concentration of transverse chemical and physical bonds eff |
10 3 |
for polymeric |
binders based on polysulfide (▲), polyester urethane (*), polyisoprene divinyl ( ), polybutadiene (○), polybutyl isoprene (●), and polydiene epoxy urethane (□). The data are transformed to the “standard” conditions: T = 293 K and = 1.4·10–3s–1
|
|
|
|
|
|
|
log 0 |
log 0 |
|
|
|
|
C |
eff |
, |
(8) |
||||
|
|
|
|
|
|
|
b |
b |
Mc |
|
|
|
|
|
|
|||||
where |
log 0 |
|
|
|
3,1 corresponds |
to |
0 |
|
|
|
|
1250 % ; C = 40, and |
||||||||
|
|
|
|
|
|
|
||||||||||||||
Mc |
Mc c |
|||||||||||||||||||
|
|
|
b |
|
|
|
|
|
|
b |
|
|
||||||||
|
|
c 1 |
according to Eq. (7). After some algebraic transformations, we have |
|||||||||||||||||
eff d / M |
||||||||||||||||||||
|
|
|
|
|
|
|
|
bo 103,1 40 |
|
eff . |
|
|
(9) |
140