
книги / Структурно-механические свойства эластомерных композитных материалов
..pdf
жД
1 2
3
a
1 |
2 |
|
|
жД |
|
|
b |
3
1
жД
2
c
3
Рис. 3. Зависимости энергии механического разрушения (W) эластомерного композита от разрывной деформации ( b ) при различных значениях объемной доли
полимера и пластификатора при температурах опыта: 1 – Т =223 К; 2 – Т=293 К
3 – Т=323 К; a –φr= 0,7 и φsw= 0,3; b – φr= 0,5 и φsw= 0,5; c – φr= 0,3 и φsw= 0,7
181
Определено, что при незначительном уменьшении энергии механического разрушения пластификация полимерной основы связующего наполненного эластомера обеспечивает повышение его эластичности при температурах эксплуатации 223…323 К в 2,5…2,2 раза.
СПИСОК ЛИТЕРАТУРЫ
1.Ermilov A.S., Nurullaev E.M. Mechanical Properties of Elastomers filled with solid Particles // Mechanics of composite Materials. 2012. Vol. 48. No. 3. PP. 243 – 252
2.Ermilov A.S., Nurullev E.M. Optimization of Fractional Composition of the Filler of elastomers Composites // Mechanics of Composite Materials. 2013. Vol. 49. No. 3. PP. 455 – 464
3.Smith T.L. Symposium on stress-strain-time-temperature relationships in materials // Amer. Soc. Test. Mat. Spec. Publ. 1962. No. 325. PP. 60–89
4.Smith T.L. // J. Appl. Phys. 1964. No. 35. PP. 27 – 32
5.Смит Т.Л. Связь между строением эластомеров и их прочностью при растяжении. В книге «Механические свoйства новых материалов» / пер. с англ. под ред. Г.И. Баренблатта. М.: Мир, 1966. – С. 174-190.
6.Smith T.L., Chy W.H. Ultimate Tensile Properties of Elastomers // J. Polymer Sce. 1972. Part A – 2. Vol. 10. No. 1. PP. 133 – 150
7.Ermilov A.S and Nurullaev E.M. // Russian Journal of Applied Chemistry, 2014, Vol. 87, No. 4, pp. 500−508
8.Патент № 2473581 РФ. Гидроизоляционное морозостойкое покрытие асфальта автомобильной дороги / Ермилов А.С., Нуруллаев Э.М., Аликин В.Н. – Приоритет от 31. 05. 2011 г.
9.Забродин В.Б., Зыков В.И., Чуй Г.Н. Молекулярная структура сшитых полимеров // Высокомолекулярные соединения. 1975.Т. (А) XVII. № 1. С. 163 -169
10.Ван Кревелен Д.В. Свойства и химическое строение полимеров / пер. с англ. под ред. А.Я. Малкина. М.: Химия, 1976. 415 с
11.Нильсен Л.Е. Механические свойства полимеров и полимерных композиций / пер. с англ. под ред. П.Г. Бабаевского. М.: Химия, 1978. – 311 с.
12.Мэнсон Д.А., Сперлинг Л.Ч. Полимерные смеси и композиты.
М.: Химия, 1979. 440 с.
182
13.Ермилов А.С., Нуруллаев Э.М., Дурегин К.А. Свидетельство
№2012613349 РФ. Программное обеспечение определения и оптимизации плотности упаковки твёрдых дисперсных наполнителей полимерных композиционных материалов (Реология) /Приоритет от 09.04.2012 г.
14.Ермилов А.С., Нуруллаев Э.М., Субботина Т.Э., Дурегин К.А. Свидетельство № 2011615640 РФ Математическое программное обеспечение прогнозирования физико-механических характеристик наполненных эластомеров. (Эластомер) / Приоритет от 18.07.2011
15.Ермилов А.С., Нуруллаев Э.М. Научные основы создания моро- зо-гидроустойчивого покрытия. Физико-химические исследования в области создания морозо-гидроустойчивого покрытия асфальта автомо-
бильных дорог Gamburg: LAP LAMBERT Academic Publishing GmbH & Co. KG, P. 2012
Mechanics of Composite Materials. 2016, Vol. 52, No. 2
OPTIMIZING THE COMPOSITION OF ELASTOMECOMPOSITES
FOR THE FRACTURE ENERGY
E. M. Nurullaev and A. S. Ermilov
Keywords: filled elastomer, fracture energy, parameter of composition, molecular structure, three-dimensional cross-linked polymer
On the basis of a computer program developed, optimization of the main parameters of the composition and molecular structure of a threedimensionally cross-linked elastomer composite for the fracture energy in uniaxial tension is investigated. By a numerical simulation – varying the structural parameters and molecular structure – the maximum value of fracture energy is found (the direct problem); for a given value of fracture energy, the required parameters of the composition are determined (the inverse problem). The solutions to the problems considered can be used in the engineering practice in creating frost-resistant moisture-proof coatings and expansion joints of asphalt highways.
183
INTRODUCTION
The parameters of composition and molecular structure are of critical importance in formation of the mechanical properties of three-dimensionally cross-linked elastomers filled with rigid particles. They are widely employed in the rubber products of mechanical engineering, shipbuilding, aircraft construction, and civil engineering. The legendary success of automobile tires is explained by the polymeric base of rubber – a three-dimensionally crosslinked rubber being in a highly elastic state over a wide range of operation temperature. An urgent problem today is the creation of rolled frost-resistant and moisture-proof coverings and materials for the expansion joints of asphalt highways located in zones with a sharply continental climate. In [1], the relation between the mechanical properties of an elastomer with a disperse filler and the above-mentioned parameters was investigated theoretically and experimentally. The application of mathematical methods for optimization of the composition of a composite being developed reduces the prices of its industrial production at the expense of minimization of the volume content of the polymer binder as a more expensive component compared with the filler, for example, silica (quartz sand). Finally, the optimum structure of a composite provides the best mechanical characteristics for it and, accordingly, an increased operating life.
As a criterion of optimization of the composition of an elastomer composite, in the computer program developed by us, we chose the energy (work) of mechanical destruction of the material in the conditions of uniaxial tension as the most widespread test method. The derivation of a calculation formula for the fracture energy, in relation to the key parameters of an elastomer composite, for the first time, was described in [2].
The purpose of the present study was to investigate, using the method of numerical simulation, the effect of parameters of the composition and molecular structure of an elastomer composite on the maximum energy of mechanical destruction (the direct problem) and to determine the necessary parameters ensuring a required value for the fracture energy (the inverse problem). The case of absence of continuity violation in the composite material up to its fracture was considered. Exactly this case is of practical interest in solving the problem of multiply increasing the service life of highways operating in the conditions of the destructive influence of repeating water-ice phase transitions. The nonlinear mathematical programming [3-5] and modern practical methods of optimization [6, 7] were used.
184

THE MAXIMUM FRACTURE ENERGY AS A FUNCTION
OF STRUCTURAL PARAMETERS
The energy of mechanical fracture W of an elastomer composite as a function of the ultimate stretch ratio αb was calculated from the formula [2]
|
|
|
|
|
|
|
|
|
|
|
|
|
|
2 |
|
|
|
|
|
|
|
|
|
|
|
|
W |
13 RT 1 1,25 |
|
m |
|
|
|
|
|
|
|
|
|
|
|
||||
|
|
|
|
|
|
|
|
|
|
|
|
|
|
|
|
|||||||
|
|
|
|
|
ch |
|
|
|
1 |
|
|
|
|
|
|
|
|
|
|
|||
|
|
|
|
|
r |
|
|
|
|
|
|
|
|
|
|
|
|
|
|
|
|
|
|
|
|
|
|
|
|
|
|
|
|
m |
|
|
|
|
|
|
|
(1) |
|||
|
3 |
3 b 2 |
|
|
|
|
3 |
|
|
|
2 |
|
1 |
|
3 |
|
|
2 |
1 |
|
||
|
b |
29exp |
10 |
T |
Tg |
|
|
2 b |
3 b |
|
||||||||||||
|
|
2 |
|
0,225 |
|
|
a |
|
2 |
2 |
|
|
||||||||||
|
|
b |
|
|
|
|
|
|
|
|
|
|
|
|
|
b |
|
|
||||
|
|
|
|
|
|
|
|
|
|
|
|
|
|
|
|
|
|
|
According to Eq. (1), we introduce the following additional parameters: W – the fracture energy;
R – the universal gas constant; T – the equilibrium temperature at which the concentration of intermolecular (“physical”) bonds is negligibly small; φ – the volume fraction of disperse filler;
φm – the limiting volume fraction of filler, which depends on the form and fractional structure of filler particles [8]; T – the testing temperature; Tg – the structural glass-transition temperature of the polymer binder; αb – the ultimate stretch ratio; a – the coefficient of displacement rate, equal to unity if
the test is performed at the standard tension rate = 1.4·10-3 s-1 assumed in the rubber branch of science and engineering.
For a numerical simulation of the relation between the maximum fracture energy and the parameters of composition and molecular structure of a polymer composite material (PCM), a computer program was developed.
The mathematical statement of the problem of search for the maximum fracture energy, with restrictions imposed on other characteristics, can be written in the form of the following problem of nonlinear programming:
|
|
|
|
|
|
|
|
|
max |
|
||
W |
, |
, |
|
m |
|
|||||||
ch |
|
r |
|
|
|
|
|
|
||||
|
|
|
|
|
ch i |
2 10 5 |
|
|||||
|
|
|
|
|
|
|||||||
0.1 10 5 |
|
|
1 In |
(2) |
||||||||
0,3 r 1 sw |
i 0,7 |
|||||||||||
|
|
|||||||||||
0,5 m 1 |
|
|
|
|||||||||
|
|
|
||||||||||
|
|
|
|
|
|
1 |
|
|
|
|
|
185

where ch , r , and / m are the vectors of molar concentration of the trans-
verse chemical bonds, volume fraction of polymer in the binder, and the effective volume fraction of filler, respectively; φsw is the volume fraction of softener, which is associated with the volume fraction of polymer φr; φr + φsw =1; In is the set of indices for the composition; n is the number of calculation variants of compositions.
The quantity αb, which is connected with the ultimate strain ε (%) by the relation
|
b (1 b /100), |
|
was found using the transition equation |
|
|
|
bf b0 1 3 m , |
(3) |
where bf |
is the ultimate strain of the elastomer composite; b0 |
is the ultimate |
strain of the free polymer binder. The value of b0 is found from the generalized relation b0 f ( eff ) expressed as the empirical formula
b0 103.1 40 eff , |
(4) |
where νeff is the effective concentration of the transverse chemical and intermolecular bonds in the polymer binder. Its value is determined by the relation [1]
eff |
1/3 |
1 29exp |
|
10 |
3 |
(T Tg ) |
2 |
(5) |
ch r |
0.225 |
|
. |
The quantity ch / Mc was calculated employing the corresponding molecular graph [1]
|
|
c |
(R3 f32 R2 f21 R1 f12 |
R2 |
f23 |
R3)n , |
|
M |
(6) |
||||||
|
|
|
|
|
|
|
|
where the combinations of subscripts on f and R designate the current molecular fragments as the products of reactions of ith and jth antipode end functional groups of PDI-3B (R1) and SKD-KTR (R2) rubbers and the EET-1 (R3) crosslinking agent, and n is the degree of copolymerization of the these rubbers.
Upon interaction of the epoxy and carboxyl groups (linear copolymerization of SKD-CTE and PDI-3B) between the three-dimensional nodes of the cross-linking epoxy agent (EET-1) and carboxyl rubber (SKD-KTR), chemical
186
bonds are formed. For this reason, the molar concentration of the transverse chemical bonds was considered.
The solution algorithm for the problem of nonlinear programming is illustrated in Fig. 1. We investigated a three-dimensionally cross-linked elastomer based on two low-molecular rubbers with end functional groups filled with silica, which is of interest as a material of waterproof cold-resistant coverings for asphalt and expansion joints of highways. From the technological viewpoint, low-molecular rubbers are needed for this purpose, because their viscosity is low and the structural glass-transition temperatures of components of the polymer binder also have to be low [9]. Therefore, two copolymerized rubbers satisfying the purposes of this investigation – the SKD-CTE (with carboxyl groups) and PDI-3B (with epoxy groups) – were chosen. As a threedimensional cross-linking agent, we used the EET-1 aliphatic resin (with three epoxy groups), and as a softener – dibutyl phthalate, thermodynamically mixable with the rubbers used.
Table 1. Characteristics of the Fractions of Silica Particles
Fraction |
Porosity (volume |
Factor |
Weight-average size |
|
fraction of pores) |
of porosity |
of particles, ϻm |
1 (fine) |
0.450 |
0.818 |
1 |
2 (average) |
0.384 |
0.623 |
30 |
3 (coarse) |
0.379 |
0.610 |
600 |
DETERMINATION OF THE MAXIMUM FRACTURE ENERGY OF A FILLED ELASTOMER (THE DIRECT PROBLEM)
The optimization algorithm developed on the basis of nonlinear programming for calculating the fractional structure of disperse filler particles of a polymer composite was applied to an elastomer material of a frost-resistant and moisture-proof rolled covering for the asphalt of highways so that to considerably improve its operating life at real temperatures, from 223 to 323 K, which was taken into account upon optimizati on.
Table 1 presents characteristics of the fractions of silica as a disperse filler of an elastomer binder based on three-dimensional cross-linked lowmolecular rubbers (oligomers) with epoxy (polydiene urethane epoxide of trademark PDI-3B) and carboxyl (polybutadiene of trademark SKD-CTE) end groups. The cross-linking agent was the EET-1 three-functional epoxy resin.
187
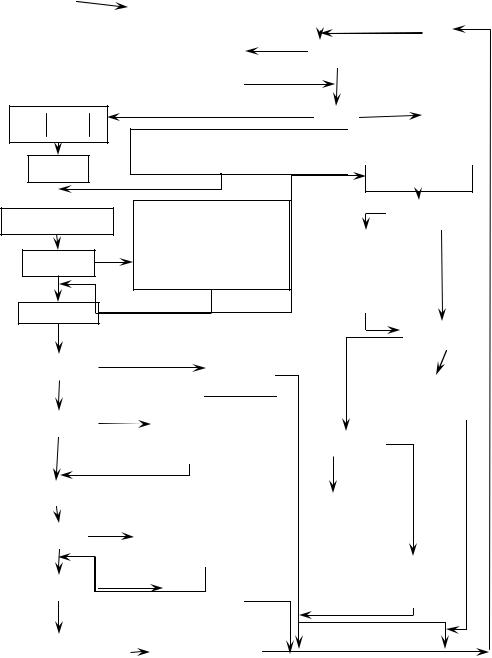
|
|
|
|
Choose: |
x 0 |
, n, α, β, |
γ, ε и Ф 0 . |
|
|
|
|
|
|
|
|
|
|
|
start |
|
|
xl 0 , f xh0 , |
f xg0 , f xl0 . |
|
|
||||||||
|
|
|
|
|
i 1, , |
n 1 , xh0 , |
|
|
|
|||||||
|
|
|
|
Find: xi0 |
xg0 , |
|
|
|||||||||
|
|
|
|
|||||||||||||
|
|
|
|
|
|
|
|
|
|
|
|
|
|
|
|
k=k+1 |
|
|
|
|
|
|
|
|
|
|
|
|
|
|
|
||
Minimize |
T xhk , to satisfy the condition T xis 0. |
|
|
|
|
|
k |
0 ? |
|
|
|
|||||
Put: x hk |
xis |
. Compute: f xhk . |
|
|
|
|
|
|
|
|
T xh |
|
|
|||
|
|
|
|
|
|
|
|
|
|
|
|
|
||||
Найти: xhk , xgk , xl k , f xhk , f xgk , f xl k . |
|
|
|
|
|
|
|
|
No |
|||||||
|
|
|
|
|
|
|
|
|
|
|
|
|
|
|
|
|
1 r 1
xo k r i 1xik xlk
Yes
T xo k 0 ? No
xr k xo k xo k xl k
Yes
T xr k 0 ?
No
f xr k f xhk ?
No |
|
|
|
|
|
|
|
Yes |
|
|
|
|
Ф |
0 |
|
ε? |
|
Stop |
|
||||
|
|
|
|
|
|
||||||
|
|
|
|
|
|
|
|
|
|||
|
|
|
|
|
|
|
|
|
|
|
|
Minimize T xo k , to satisfy the condition |
T xis 0 . |
|
|
|
|
|
|
|
|||
Put: xo k xis . |
|
|
|
|
|
|
|
|
|
|
|
|
|
|
|
|
|
|
xe k |
γ xr k 1 γ xo k |
|
||
|
|
|
|
|
|
|
|
|
Minimize |
T xr k , to satisfy the condi- |
|
tion |
T xis 0. |
|
Put: |
xr k |
xis . |
Compute: f xr k .
Yes
|
Yes |
|
|
|
|
|
T xe k 0 ? |
|
|
||
|
|
|
|
||
|
|
|
|
|
|
|
|
|
|
||
Minimize |
T xe k , to satisfy the |
|
|
|
|
condition |
T xis 0 . |
|
No |
||
|
|
|
|
||
Put: xe k |
xis . |
|
|
|
|
Compute: |
f xe k . |
|
|
|
|
|
|
|
|
|
|
|
|
|
|
|
No |
|
|
|
|
|
|
|
|
|
|
|
|
|
|
|
|
|
|
|
|
|
|
|
|
|
|
|
|
|
|
|
|
|
|
|
|
|
|
f xe k f xhk ? |
||||
|
|
|
|
|
|
|
|
|
|
|
|
|
|
|
|
|
No |
|
|
|
|
|
|
|
|
|
|
|
|
|
|
|
|
|
|
|
|
|
|
|
|
|
|
|
|
|
||
|
|
|
|
|
|
|
|
|
|
|
|
|
|
|
|
|
|
|
|
|
|
|
|
|
|
|
|
|
|
|
|
|
|
|
|
|
|
|
|
|
|
No |
|
|
|
|||
|
|
|
f |
xr k |
|
f |
|
x k |
|
? |
|
|
|
|
|
|
|
|
|
Put: |
|
|
|
|
|
|
|
|
|
|
|
|
|
|
|
|
|
|
|
|||||||||
|
|
|
|
|
|
|
|
|
|
|
|
|
|
|
|
|
|
|
|
|
|
|
|
|
|
|
|
|
|
|
|
|
|
|
|
|
||||||||||||
|
|
|
|
|
|
|
|
g |
|
|
|
|
|
|
|
|
|
|
|
|
|
|
x |
|
|
xr |
, |
|
|
|
|
|
|
|
|
|
|
|
|
|
|
|
|
|||||
|
|
|
|
|
|
|
|
|
|
|
|
|
|
|
|
|
|
|
|
|
. |
|
|
Yes |
|
|
|
|
|
|
|
|
Put: xl k |
xr k , |
|
|||||||||||||
|
|
|
|
Yes |
|
|
|
|
|
|
|
|
|
|
|
|
|
|
|
|
|
|
|
|
|
|
|
|
|
|
|
|
|
|
||||||||||||||
|
|
|
|
|
|
|
|
|
|
|
|
|
|
Yes |
|
|
|
|
|
|
|
f x |
f xr |
|
|
|
|
|
|
|
|
|
|
|
|
|
f xl k f xr k . |
|
||||||||||
|
|
f xr k f xl k ? |
|
|
|
Put: |
k |
xr |
k |
|
|
|
|
|
|
|
|
|
|
|
|
|
|
|
|
|
|
|
|
|
|
|
|
|||||||||||||||
|
|
|
|
|
|
|
|
|
|
|
|
|
|
|
|
|
|
xl |
|
|
|
, |
|
|
|
|
|
|
|
|
f xe k f xr k ? |
|
|
|
|
|
|
|
||||||||||
|
|
|
|
|
No |
|
|
|
|
|
|
|
|
|
|
|
f xl k f xr k . |
|
|
|
|
|
|
|
|
|
|
|
|
|
||||||||||||||||||
|
|
|
|
|
|
|
|
|
|
|
|
|
|
|
|
|
|
|
|
|
|
|
|
|
|
|
|
|
|
|
|
|
|
|
|
|
Да |
|
|
|
|
|
|
|
|
|
||
|
|
|
|
|
|
|
|
|
|
|
|
|
|
|
|
|
|
|
|
|
|
|
|
|
|
|
|
|
|
|
|
|
|
|
|
|
|
|
|
|
|
|
|
|
|
|||
|
|
|
|
|
|
|
|
|
|
|
|
|
|
|
|
|
|
|
|
|
|
|
|
|
|
|
|
|
|
|
|
|
|
|
|
|
|
|||||||||||
|
xc k |
β xlk 1 β xo k . |
|
|
|
Minimize |
T xc k , |
|
to satisfy the |
|
|
|
|
Put: |
|
|
|
|
|
|
|
|
|
No |
|
|
|
|||||||||||||||||||||
|
|
|
|
|
|
|
|
|
|
|
|
|
|
|
|
|
condition |
T xis 0. |
|
|
|
|
|
|
|
|
|
xl k |
xe k , |
|
|
|
|
|
|
|
|
|
||||||||||
|
|
|
|
|
|
|
|
|
|
|
Yes |
|
|
|
|
|
|
|
|
|
|
|
|
|
|
|
|
|
|
|
|
|
|
k |
f |
xe |
k |
. |
|
|
|
|
|
|||||
|
|
|
|
T xc k |
0? |
|
|
|
|
|
|
|
Put: xc k |
xis . |
|
|
|
|
|
|
|
|
|
|
|
|
f xl |
|
|
|
|
|
|
|||||||||||||||
|
|
|
|
|
|
|
|
|
|
|
|
|
|
|
|
Compute: f xc k . |
|
|
|
|
|
|
|
|
|
|
|
|
|
|
|
|
|
|
|
|
|
|
|
|
||||||||
|
|
|
|
|
No |
|
|
|
|
|
|
|
|
|
|
|
|
|
|
|
|
|
|
|
|
|
|
|
|
|
|
|
|
|
|
|
|
|
|
|||||||||
|
|
|
|
|
|
|
|
|
|
|
|
|
|
|
|
|
|
|
|
|
|
|
|
|
|
|
|
|
|
|
Put: |
|
|
|
||||||||||||||
|
|
|
|
|
|
|
|
|
|
|
|
|
|
|
|
|
|
|
|
|
|
|
|
|
|
|
|
|
|
|
|
|
|
|
|
|
|
|
|
|
|
|
|
|||||
|
|
|
|
|
|
|
|
|
|
|
|
|
|
|
No |
|
|
|
|
|
|
|
|
|
|
|
|
|
|
|
|
|
|
|
|
|
|
|
|
xl k xr k , |
|
|
|
|||||
|
|
f xc |
k |
f |
|
k |
? |
|
|
|
|
Put: |
|
|
|
|
|
|
|
|
|
|
|
|
|
|
|
|
|
|
|
|
|
|
|
|||||||||||||
|
|
|
|
|
|
|
|
|
|
|
|
|
|
|
|
|
|
|
|
|
|
|
|
|
|
|
|
|
|
|
|
|
|
|
|
|
|
|||||||||||
|
|
|
xl |
|
|
|
|
|
|
|
xl k |
xc k , |
|
|
|
|
|
|
|
|
|
|
|
|
|
|
|
|
f xl k f xr k . |
|
||||||||||||||||||
|
|
|
|
Yes |
|
|
|
|
|
|
|
|
|
|
|
|
f xl k f xc k . |
|
|
|
|
|
|
|
|
|
|
|
|
|
|
|
|
|
|
|
|
|||||||||||
|
|
|
|
|
|
|
|
|
|
|
|
|
|
|
|
|
|
|
|
|
|
|
|
|
|
|
|
|
|
|
|
|
|
|||||||||||||||
|
|
|
|
|
|
|
|
|
|
|
|
|
|
|
|
|
|
|
|
|
|
|
|
|
|
|
|
|
|
|
|
|
|
|
|
|
|
|
|
|
|
|
|
|
|
|||
|
|
|
|
|
|
|
|
|
|
|
|
|
|
|
|
|
|
|
|
|
|
|
|
|
|
|
|
|
|
|
|
|
|
|
|
|
|
|||||||||||
Determine: |
|
|
|
|
|
|
|
|
|
|
|
|
|
|
Determine new values: |
|
|
|
|
|
|
|
|
|
|
|
|
|
|
|
|
|
|
|
|
|||||||||||||
xik 0.5 |
xik xlk , |
i 1, , |
|
|
|
|
f xik ;i 1, , |
|
|
|
|
|
|
|
|
|
|
|
|
|
|
|
|
|
|
|
|
|
|
|||||||||||||||||||
r 1 |
|
|
|
r 1 |
|
|
|
|
|
|
|
|
|
|
|
|
|
|
|
|
|
|
|
|
||||||||||||||||||||||||
|
|
|
|
|
|
|
|
|
|
|
|
|
|
|
|
|
|
|
|
|
|
|
|
|
|
|
|
|
|
|
|
|
|
|
|
|
|
|
|
|
|
|
|
|
|
|
|
|
Fig. 1. Solution algorithm for the problem of nonlinear programming.
188
Table 2. Maximum Values of the Fracture Energy
at Various Polymer/Softener Ratios
Varied parameters of the composition |
Maximum values |
|||
and molecular structure of the elastomer composite |
of characteristics |
|||
Т, К |
νch, mol/m3 |
φ/φm |
εb, % |
Wb, МJ |
|
|
0.7/0.3 |
|
|
223 |
5 |
0.90 |
15 |
1.85 |
273 |
10 |
0.87 |
20 |
1.79 |
293 |
30 |
0.85 |
25 |
1.75 |
323 |
50 |
0.87 |
30 |
1.45 |
|
|
0.5/0.5 |
|
|
223 |
5 |
0.90 |
18.05 |
1.76 |
273 |
10 |
0.87 |
23.49 |
1.71 |
293 |
30 |
0.85 |
28.31 |
1.67 |
323 |
50 |
0.87 |
32.62 |
1.33 |
|
|
0.3/0.7 |
|
|
223 |
0.5 |
0.90 |
19.41 |
1.69 |
273 |
1.0 |
0.87 |
25.32 |
1.64 |
293 |
3.0 |
0.85 |
30.42 |
1.59 |
323 |
5.0 |
0.87 |
34.18 |
1.21 |
N o t e: a = 1.0.
The influence of the effective volume fraction of filler φ/φm = const at on the energy of mechanical destruction of the elastomer composite was estimated with the use of silica specimens of the following fractional compositions: 1 – the initial (control) specimen – 600 μm : 30 μm = 20 : 80 and 2 – an experimental (optimum) specimen – 600 μm : 30 μm : 1 μm = 50 : 30 : 20.
The specific contact surface between the particles and binder was regarded as the quantity inverse to the size of particles (it was about the same in both cases of filler mixtures).
Table 2 shows the results of numerical simulation of search for the maximum value of energy of mechanical destruction of the material ensuring an increased service life of the coverings and expansion joints of asphalt of highways. Considering the thermodynamic compatibility and technological properties of the elastomer composite, the following polymer/softener ratios were chosen: 0.7/0.3, 0.5/0.5, and 0.3/0.7. Taking into account the required mechan-
189
ical characteristics and technological possibilities, the molar concentration of the transverse chemical bonds was varied in the range from 5 to 50 mol/m3. As seen from data of the Table, the maximum value of energy of mechanical destruction of the composite was achieved at the maximum content of the polymeric base of the binder. In this case, a small decrease in the ultimate strain was observed.
CALCULATION OF THE COMPOSITION OF A COMPOSITE ACCORDING TO A GIVEN VALUE OF FRACTURE ENERGY (THE INVERSE PROBLEM)
The parameters of composition and molecular structure of a polymer composite, which, as shown by practical operation of platforms of sports constructions, stipulate the necessary energy and ultimate strain (the inverse problem), were determined at W = 1.1-1.7 MJ and εb = 70-20%. The case of absence of continuity violation in the composite material up to its rupture was considered. The data are reflected in Table 3.
|
Table 3. Inverse Problem at Different Values of W and εb |
|
||||||
|
|
|
|
|
|
|
||
Required parameters |
Calculated parameters of the composition |
|||||||
of the elastomer composite |
|
and molecular structure |
|
|||||
Т, К |
W, МJ |
εb, % |
φr |
|
ν ch, |
φ/φm |
|
φsw |
|
|
|
|
|
mol/m3 |
|
|
|
|
|
W = 1.1- |
1.4 МJ. εb = 50-20% |
|
|
|
||
223 |
1.4 |
20 |
0.31 |
|
2.5 |
0.92 |
|
0.69 |
273 |
1.3 |
30 |
0.3 |
|
7.9 |
0.88 |
|
0.7 |
293 |
1.2 |
40 |
0.45 |
|
12.4 |
0.84 |
|
0.55 |
323 |
1.1 |
50 |
0.3 |
|
27 |
0.82 |
|
0.7 |
|
|
W = 1.1- |
1.7 МJ. εb = |
70-25% |
|
|
|
|
223 |
1.7 |
25 |
0.70 |
|
5.7 |
0.84 |
|
0.30 |
273 |
1.5 |
35 |
0.30 |
|
13.8 |
0.82 |
|
0.70 |
293 |
1.3 |
45 |
0.40 |
|
17.0 |
0.81 |
|
0.60 |
323 |
1.1 |
70 |
0.36 |
|
29.1 |
0.74 |
|
0.7 |
|
|
W = 1.1- |
1.4 МJ. εb = 30-15% |
|
|
|
||
223 |
1.4 |
15 |
0.32 |
|
0.22 |
0.94 |
|
0.68 |
273 |
1.3 |
20 |
0.40 |
|
0.60 |
0.92 |
|
0.60 |
293 |
1.2 |
25 |
0.58 |
|
1.01 |
0.90 |
|
0.42 |
323 |
1.1 |
30 |
0.30 |
|
2.26 |
0.89 |
|
0.70 |
190