
книги / Структурно-механические свойства эластомерных композитных материалов
..pdf
при принятых ранее обозначениях применительно к уравнению (1) происходит в меньшей степени. Этим объясняется соответствующее возрастание значения W и, следовательно, абсолютной энергии механического разрушения эластомерного композита.
Wr
αb
Рис. 2. Расчетная зависимость энергии механического разрушения W наполненного трехмерно сшитого эластомера от относительного разрывного удлинения αb. Температура эксперимента Т = 293 К; концентрация поперечных
химических связей в связующем νch = 1·10–5 моль/см3
Полученную на теоретической основе зависимость (5) энергии механического разрушения трехмерно сшитого наполненного эластомера от основных структурных параметров композита можно рекомендовать для решения прямой и обратной задач при проектировании новых полимерных композитов различного назначения с требуемым комплексом эксплуатационных характеристик [1]. При этом целесообразно пользоваться компьютерными программами, включающими математические методы оптимизации [2, 15, 16]. Естественно, что это приведет к сокращению сроков разработки и экономии сырья, материалов, например, при создании гидроизоляционного морозостойкого покрытия асфальта автомобильных дорог [10, 17].
151
ЗАКЛЮЧЕНИЕ
Таким образом, в настоящей работе на физико-математической основе получена формула, связывающая энергию механического разрушения (в условиях одноосного растяжения) наполненного трехмерно сшитого эластомера и его основные структурные параметры. Предложена обобщенная зависимость относительного разрывного удлинения полимерного связующего от эффективной концентрации химических и физических (межмолекулярных) связей, необходимая при проектировании новых композитов.
СПИСОК ЛИТЕРАТУРЫ
1.Ermilov A.S. and Nurullaev E.M. Mechanical properties of elastomers filled with solid particles // Mech. Compos. Mater. – 2012. – Vol. 48, No. 3. – P. 243–252.
2.Ermilov A.S. and Nurullev E.M. Optimization of fractional composition of the filler of elastomer composites // Mech. Compos. Mater. – 2013. – Vol. 49, No. 3. – P. 311–316.
3.Smith T.L. Symposium on stress-strain-time-temperature relationships in materials // Amer. Soc. Test. Mat. Spec. Publ. – 1962. – No. 325. – P. 60–89.
4.Smith T.L. Limited characteristics of crosslink polymers // J. Appl. Phys. – 1964. – No. 35. – P. 27–32.
5.Смит Т.Л. Связь между строением эластомеров и их прочностью
при растяжении // Механические свoйства новых материалов / пер.
сангл. под ред. Г.И. Баренблатта. – М.: Мир, 1966. – С. 174–190.
6.Smith T.L. and Chy W.H. Ultimate tensile properties of elastomers // J. Polymer Sci. – 1972. – Pt A2. – Vol. 10, No. 1. – P. 133–150.
7.Ermilov A.S. and Fedoseev A.M. Combinatorial-multiplicative method of calculating the limiting filling of composites with solid dispersed components // J. of Appl. Chem. – 2004. – Vol. 77, No. 7. – P. 1203–1205.
8.Ермилов А.С., Нуруллаев Э.М. Концентрационная зависимость усиления каучуков и резин дисперсными наполнителями // Журн. прикл.
химии. – 2012. – Т. 85, № 8. – С. 1371–1374.
9.Бронштейн И.Н., Семендяев К.А. Справочник по математике для инженеров и учащихся ВТУЗов. – М: Наука, 1986. – 544 с.
152
10.Патент № 2473581 РФ. Гидроизоляционное морозостойкое покрытие асфальта автомобильной дороги / А.С. Ермилов, Э.М. Нуруллаев, В. Н. Аликин. – Приоритет от 31. 05. 2011 г.
11.Забродин В.Б., Зыков В.И., Чуй Г.Н. Молекулярная структура сшитых полимеров // Высокомолекуляр. соединения. Сер. А. – 1975. –
Т. XVII, № 1. – С. 163–169.
12.Ван Кревелен Д.В. Свойства и химическое строение полимеров / пер. с англ. под ред. А.Я. Малкина. – М.: Химия, 1976. – 415 с.
13.Нильсен Л.Е. Механические свойства полимеров и полимерных композиций / Пер. с англ. под ред. П.Г. Бабаевского. – М.: Химия, 1978. – 311 с.
14.Мэнсон Д.А., Сперлинг Л.Ч. Полимерные смеси и композиты / Пер. с англ. под ред. Ю.К. Годовского. – М.: Химия, 1979. – 440 с.
15.Свидетельство № 2012613349 РФ. Программное обеспечение определения и оптимизации плотности упаковки твердых дисперсных наполнителей полимерных композиционных материалов (реология) / А.С. Ермилов, Э.М. Нуруллаев, К.А. Дурегин. – Приоритет от
09.04.2012 г.
16.Свидетельство № 2011615640 РФ. Математическое программное обеспечение прогнозирования физико-механических характеристик наполненных эластомеров (эластомер) / А.С. Ермилов, Э.М. Нуруллаев. Т.Э. Субботина, К.А. Дурегин. – Приоритет от 18.07.2011 г.
17.Ермилов А.С., Нуруллаев Э.М. Научные основы создания моро-
зо-гидроустойчивого покрытия. Физико-химические исследования в области создания морозо-гидроустойчивого покрытия асфальта авто-
мобильных дорог. – Germany, Gamburg: LAP LAMBERT Academic Publishing GmbH & Co. KG, 2012. – 223 S.
153
Russian Journal of Applied Chemistry, 2015, Vol. 88, No. 1
INFLUENCE OF PHYSICOCHEMICAL PARAMETERS ON THE ENERGY OF MECHANICAL FAILURE OF AN ELASTOMER
FILLED WITH POLYFRACTIONAL SILICON DIOXIDE
E. M. Nurullaev and A. S. Ermilov
Perm National Research Polytechnic University
ABSTRACT–The influence of the effective concentration of cross-links, including permanent chemical bonds and variable intermolecular (physical) contacts, of the fractional composition of the dispersed filler, and of plasticization on the energy of the mechanical failure of a three-dimensionally cross-linked plasticized elastomer reinforced with silicon dioxide was examined. The computer program developed by the authors was used for calculating the energy of the mechanical failure of real composites as applied to the problem of the development of a wearresistant polymeric composite material for waterproofing frost-resistant asphalt coating and deformation seams.
Polymeric composite materials based on a threedimensionally crosslinked elastomeric matrix filled with solid particles are widely used in various parts and units in aviation, ship, and automobile building. Elastomers filled with polyfractional silicon dioxide are used in building industry, including house roofi ng and flooring in sports facilities.
The mechanical characteristics of these materials largely determine the operation life of a specifi part or unit. The most important physicochemical parameters are the molecular structure of the chain in the polymer base of the plasticized binder and the volumetric degree of effective fi lling, which depends on the shape and fractional composition of dispersed fi ller particles and on the intermolecular interaction at the elastomer–filler interface [1]. Knowledge of the relationship between the structural and mechanical characteristics allows solution of both direct and inverse optimization problems in the development of new polymeric composites with the required set of operation properties [2].
When determining the mechanical characteristics at uniaxial extension, as the most frequent deformation in engineering practice, it is appropriate to plot envelopes of the specimen failure points determined at different temperatures and strain velocities according to Smith [3–6] in the coordinates
154
log (σbT0/T)–log (αb – 1), where σb and αb are the breaking stress and relative elongation at break, respectively; T and T0 are the testing temperature and the chosen reference temperature (usually 293 K).
Thus, the envelope of the specimen failure points is indirectly related to the area under the extension diagram, i.e., to the energy (work) of mechanical failure of a polymeric composite. This fact, however, does not allow more complete evaluation of the mechanical characteristics of polymeric composite materials (PCMs). An equation for the failure energy of a filled elastomer was derived previously [7]; its use allows unambiguous estimation of the infl uence of physicochemical parameters on the mechanical characteristics of PCMs.
In this study we examined by the numerical method the infl uence of the main physicochemical parameters onthe mechanical failure energy determining the operation life of a three-dimensionally cross-linked plasticized elastomer fi lled with polyfractional silicon dioxide.
We believe that the PCM for waterproofi ng frostresistant asphalt coating and deformation seams of road should be based on low-molecular-mass rubbers, because they have low viscosity. In addition, components (polymers, plasticizer) of the polymeric binder (matrix) should have low structural glass transition points [8].
The characteristics of some rubbers with terminal functional groups, used for three-dimensional crosslinking, are given in Table 1. As can be seen, the most suitable materials for solving the problem under consideration are commercial rubbers of grades SKD – KTR and PDI-3B. It is appropriate to use EET-1 trifunctional epoxy resin as cross-linking agent.
Table 1. Characteristics of some rubbers with terminal functional groups
Rubber grade |
Content of |
Viscosity at |
Weight-average |
Glass transi- |
||
|
functional |
50°C, Pa s |
molecular mass |
tion point |
||
|
groups, % |
|
|
|
s 103 |
Tg, °C |
|
|
М |
||||
SKD -NK |
- |
900 |
45 |
|
|
-103 |
SKD-NL |
- |
1200 |
ПО |
-70 |
||
SKD-1 |
3-3,5 |
40-90 |
5 - 7 |
|
|
-70 |
SKN-10-1 |
2,5-2,8 |
80-120 |
4 - 7 |
|
|
-60 |
SKN -18-1 |
2,5-2,8 |
100-140 |
4 - 7 |
|
|
-55 |
SKN -26-1 |
2,5-2,8 |
40 |
4 - 7 |
|
|
-38 |
SRD-KTR |
2,0-2,4 |
20 (25 °С) |
3 |
|
|
-78 |
-SKN- 10KTR |
2,9-3,1 |
30(25 °С) |
3 |
|
|
-60 |
SKD-GTR |
0,9-1,4 |
10(25°С) |
3 |
|
|
-70 |
PDI-ЗB |
1,6-2,1 |
15-22 |
4 |
|
|
-80 |
155

Scheme 1.
|
|
|
|
|
|
|
|
|
|
|
|
|
|
|
|
|
|
|
|
|
|
|
|
|
|
|
|
HC |
|
|
CH2 |
|
HOOC |
HOOC |
|
|
R1 |
|
|
COOH + H2C |
|
|
CH |
|
|
|
R2 |
|
|
|
|
||||||||||||||||
|
|
|
|
O |
|
|
|
|
|
|
|
|
|||||||||||||||||||||
|
|
|
|
|
|
||||||||||||||||||||||||||||
SKD-KTR rubber |
|
|
|
|
|
|
|
|
|
|
|
|
|
|
|
O |
|
|
|||||||||||||||
|
|
|
|
PDI-3B rubber |
|
|
|
|
|
|
|
||||||||||||||||||||||
|
|
R |
1 |
|
|
C |
|
O |
|
CH |
2 |
|
CH |
|
|
R2 |
|
HC |
|
CH |
2 |
|
|
|
|
||||||||
|
|
|
|
|
|
|
|
|
|
|
|
|
|
|
|||||||||||||||||||
|
|
|
|
|
|
|
|
|
|||||||||||||||||||||||||
|
|
|
|
|
|
|
|
|
|
|
|
|
|
|
|
|
|
|
|
|
|
|
|
|
|
|
O |
|
|
|
|
|
|
|
|
|
|
|
|
O |
|
|
|
|
|
|
OH |
|
|
|
|
|
|
|
|
|
|
|
|
Here and hereinafter, Ri denotes the molecular chain of 3D network components, with i = 1 referring to SKD-KTR; i = 2, to PDI-3B; and i = 3, to EET-1.
Scheme 2.
COOH |
|
|
CH2 |
|
|
CH |
|
|
|
|
|
|
|
|
|
|
|
|
+ HOOC |
||||||||||||||
|
|
|
|
|
|
|
|
|
|
|
|
|
|||||||||||||||||||||
|
|
|
|
|
|
+ |
|
|
|
O |
|
|
R |
|
|
|
CH |
|
CH2 |
||||||||||||||
|
|
|
|
|
|
|
|
|
|
|
|
||||||||||||||||||||||
|
|
|
|
|
|
|
|
3 |
|
||||||||||||||||||||||||
|
|
|
|
|
|
|
|
|
|
|
|
|
|
|
|
|
|
|
|
|
|
|
|
|
|
|
|
|
|
|
|
||
|
|
|
|
|
|
|
|
|
|
|
|
|
|
|
|
|
|
|
|
|
|
|
|
|
|
O |
|
|
|
|
|||
COOH |
|
|
CH2 |
CH |
|
|
|
|
|
|
|
|
|
|
|||||||||||||||||||
|
|
|
|
|
|
|
|
|
|
|
|
|
|
|
|
|
|
||||||||||||||||
|
|
|
|
|
|
|
|
|
|
|
|
O |
|
|
EET-1 epoxy resin |
||||||||||||||||||
|
|
|
|
|
|
|
|
|
|
|
|
|
|
|
|
|
|
|
|
|
|
|
|
|
|
|
|
|
|
||||
O |
|
|
|
|
|
|
OH |
|
|
|
|
|
|
|
|
|
|
|
|
|
|
|
|
|
|
||||||||
|
|
|
|
|
|
|
|
|
|
|
|
|
|
|
|
|
|
|
|
|
|
|
|
O |
|||||||||
|
|
|
|
|
|
|
|
|
|
|
|
|
|
|
|
|
|
|
|
|
|
|
|
|
|
|
|
|
|
|
|||
|
|
|
|
|
|
|
|
|
|
|
|
|
|
|
|
|
|
|
|
|
|
|
|
|
|
|
|
|
|
|
|
|
|
C |
|
|
O |
|
|
CH2 |
CH |
|
|
|
|
|
|
|
|
|
|
|
|
|
|
|
|
|
|
||||||||
|
|
|
|
|
|
|
|
|
|
|
|
|
|
|
|
|
|
|
|
|
|
||||||||||||
|
|
|
|
|
|
|
|
|
|
|
|
|
|
|
|
R |
|
|
|
CH |
|
|
CH2 |
O |
|
C |
|||||||
C |
|
|
O |
|
|
CH2 |
|
|
|
CH |
3 |
|
|
|
|
|
|||||||||||||||||
|
|
|
|
|
|
|
|
|
|||||||||||||||||||||||||
|
|
|
|
|
|
|
|
|
|
|
|
|
|
|
|
|
|
|
|
|
|
|
|
||||||||||
|
|
|
|
|
|
|
|
|
|
|
|
|
|
|
|
|
|
|
|
|
|
|
|
|
|||||||||
|
|
|
|
|
|
|
|
|
OH |
|
|
|
|
|
|
|
|
|
|
||||||||||||||
|
|
|
|
|
|
|
|
|
|
|
|
|
|
|
|
|
|
||||||||||||||||
|
|
|
|
|
|
|
|
|
|
|
|
|
|
|
|
|
|
|
|
|
|
|
|
|
|
|
|
||||||
|
|
|
|
|
|
|
|
|
|
OH |
|
|
|
|
|
|
|
|
|
|
|
|
|
|
|
|
|
|
|||||
O |
|
|
|
|
|
|
|
|
|
|
|
|
|
|
|
|
|
|
|
|
|
|
|
|
|||||||||
|
|
|
|
|
|
|
|
|
|
|
|
|
|
|
|
|
|
|
|
|
|
|
|
|
|
|
|
|
|
|
The equation for calculating the energy of mechanical failure of a filled elastomer in relation to the main physicochemical parameters of the composition, derived in [7], has the form where νch = ρ/ is the concentration of chemical crosslinks in the polymeric base of the binder; ρ, polymer density; , statistical mean molecular mass of the chain segment between the crosslinking nodes of the 3D cross-linked polymer; φr = (1 – φsw), volume fraction of the polymer in the binder; φsw, volume fraction of the plasticizer in the binder; R, universal gas constant; T∞, equilibrium temperature at which the intermolecular interaction (concentration of “physical” bonds νph) in the polymeric binder is negligibly low (νph → 0); T, specimen testing temperature;
156
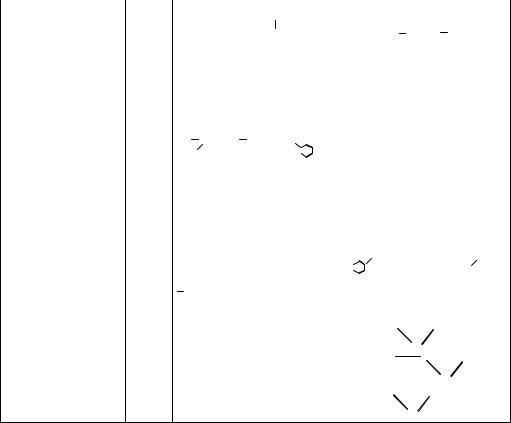
Tg, structural glass transition point of the polymeric binder; , velocity shift coeffi cient; φ, volume fraction of the filler; φm, maximum possible (limiting) volume fraction of the filler, depending on the shape and fractional composition of filler particles.
As seen from Eq. (1), the failure energy of an elastomer filled with polyfractional dispersed particles depends on many physicochemical parameters which, in turn, will determine the mechanical and other characteristics of the PCM obtained. Therefore, it is interesting to examine the infl uence of these parameters on the PCM failure energy. The failure energy W is equal to zero at αb = 1, i.e., it is a normalizable function. The limiting values of the relative elongation αb and tensile strain εb can be estimated taking into account the velocity and magnitude of the deformation of the statistical mean interlayer of thepolymeric binder between solid filler particles [1]:
Table 2. Chemical formulas of commercial butadiene rubbers
Divinyl rubber |
SKD- |
|
|
|
|
|
CH3 |
|
|
|
|
|
|
|
|
CH3 |
||||||||||||
with terminal |
KTR HOOC |
|
|
|
|
|
|
|
|
|
|
|
|
|
|
)n |
|
|
|
CH2 |
CH |
|
COOH |
|||||
|
CH |
|
CH |
|
|
C |
|
( |
|
CH |
|
CH |
|
CH |
|
CH |
|
C |
|
|
||||||||
|
|
|
|
|
|
2 |
|
|
2 |
|
2 |
|||||||||||||||||
carboxy groups |
2 |
|
|
2 |
|
|
|
|
|
|
|
|
|
|
|
|
|
|
|
|
|
|
|
|
||||
|
|
|
|
|
|
|
CN |
|
|
|
|
|
|
|
|
|
CN |
Block copolymer |
CH2 |
CH |
|
|
CH2 |
|
|
O |
|
|
|
|
C |
|
|
NH |
|
CH3 |
|
|
|
|
|
|
|
|
|
|
|
|
|
|
|
|
|
|
|
|
|
|
|
|
|
|
|
|
|
|
|
|
|
|
|
|
|
|
|
|
|
|
|
|
|
|
|||||||||||||||||||||||
|
|
|
|
|
|
|
|
|
|
|
|
|
|
|
|
|
|
|
|
|
|
|
|
|
|
|
|
|
|
|
|
|
|
|
|
|
|
|
|
|
|
|
|
|
|
|
|
|
|
|
|
|
|
||||||||||||||||||||||||||||||||||
|
|
|
|
|
|
|
|
|
|
|
|
|
|
|
|
|
|
|
|
|
|
|
|
|
|
|
|
|
|
|
|
|
|
|
|
|
|
|
|
|
|
|
|
|
|
|
|
|
|
|
|
|
|||||||||||||||||||||||||||||||||||
of divinyl and iso- |
|
O |
|
|
|
|
|
|
|
|
|
|
|
|
|
|
|
|
|
|
|
|
|
|
|
|
|
|
|
|
|
|
|
|
|
|
|
|
|
|
|
|
|
|
|
|
|
|
|
|
|
|
|
|
|
|
|
|
|
|
|
|
|
|
|
|
|
|
|
|
|
|
|
|
|
|
|
|
|
||||||||
|
|
|
|
|
|
|
|
|
|
|
|
|
|
|
|
|
|
|
|
|
|
|
|
|
|
|
|
|
|
|
|
|
|
|
|
|
|
|
|
|
|
|
|
|
|
|
|
|
|
|
|
|
|
|
|
|
|
|
|
|
|
|
|
|
|
|
|
|
|
|
|
|
|
|
|
|
|
||||||||||
|
|
|
|
|
|
|
|
O |
|
|
|
|
|
|
|
|
|
|
|
|
|
|
|
|
|
|
|
|
|
|
|
|
|
|
|
|
|
|
|
|
|
( |
|
|
|
|
CH |
|
|
|
CH |
|
|
CH |
|
|
CH |
|
) |
|
|
||||||||||||||||||||||||||
|
|
|
|
|
|
|
|
|
|
|
|
|
|
|
|
|
|
|
|
|
|
|
|
|
|
|
|
|
|
|
|
|
|
|
|
|
|
|
|
|
|
|
|
|
|
|
|
|
|
|
|
|
|
||||||||||||||||||||||||||||||||||
|
|
|
|
|
|
|
|
|
|
|
|
|
|
|
|
|
|
|
|
|
|
|
|
|
|
|
|
|
|
|
|
|
|
|
|
|
|
|
|
|
|
|
|
|
|
|
|
|
|
|
|
|
|
|
|
|
|
|
|
|
|
|
|
|
|
|
|
|
|
|
|
||||||||||||||||
|
|
|
|
|
|
|
|
|
|
|
|
|
|
|
|
|
|
|
|
|
|
|
|
|
|
|
|
|
|
|
|
|
|
|
|
|
|
|
|
|
|
|
|
|
|
|
|
|
|
|
|
|
|
|
|
|
|
|
|
|
|
|
|
|
|
|
|
m |
|
||||||||||||||||||
prene with termi- |
|
|
|
|
|
|
|
|
|
|
|
|
|
|
|
|
|
|
|
|
|
|
|
|
|
|
|
|
NH C |
O |
CH |
|
|
CH2 |
|
|
|
|
|
|
|
|
|
|
|
|
2 |
|
|
|
|
|
|
|
|
|
|
|
|
|
2 |
|
|
|
|||||||||||||||||||||||
|
|
|
|
|
|
|
|
|
|
|
|
|
|
|
|
|
|
|
|
|
|
|
|
|
|
|
|
|
|
|
|
|
|
|
|
|
|
|
|
|
|
|
|
|
|
|
|
|
|
|
|
|
|
|
|
|
|
|
|
|
|
|
|
|
|
|
|
|
|
|
|
|
|
|
|
|
|
|
|
|
|
|
|
|
|
|
|
nal epoxy groups. |
|
|
|
|
|
|
|
|
|
|
|
|
|
|
|
|
|
|
|
|
|
|
|
|
|
|
|
|
|
|
|
|
|
|
|
|
O |
|
|
|
CH3 |
CH |
|
|
|
CH |
|
|
)n |
|
|
|
|
|
|
|
|
|
|
|
|
|
|
|
|
|
|
|
|||||||||||||||||||
|
|
|
|
|
|
|
|
|
|
|
|
|
|
|
|
|
|
|
|
|
|
|
|
|
|
|
|
|
|
|
|
|
|
|
( |
|
|
CH |
|
C |
|
|
|
|
|
|
|
|
|
|
|
|
|
|
|
|
|
|
|
|
|
|
|
|
|
|
|
||||||||||||||||||||
Oligo(divinyl– |
|
|
|
|
|
|
|
|
|
|
|
|
|
|
|
|
|
|
|
|
|
|
|
|
|
|
|
|
|
|
|
|
|
|
|
|
|
|
|
|
|
|
|
|
|
|
|
|
|
|
|
|
|
|
|
|
|
|
|
|
|
|
|
||||||||||||||||||||||||
|
|
|
|
|
|
|
|
|
|
|
|
|
|
|
|
|
|
|
|
|
|
|
|
|
|
|
|
|
|
|
|
|
|
|
|
|
2 |
|
|
|
|
|
|
2 |
|
|
|
|
|
|
|
|
|
|
|
|
|
|
|
|
|
|
|
||||||||||||||||||||||||
|
|
|
|
|
|
|
|
|
|
|
|
|
|
|
|
|
|
|
|
|
|
|
|
|
|
|
|
|
|
|
|
|
|
|
|
|
|
|
|
|
|
|
|
|
|
|
|
|
|
|
|
|
|
|
|
|
|
||||||||||||||||||||||||||||||
|
|
|
|
|
|
|
|
|
|
|
|
|
|
|
|
|
|
|
|
|
|
|
|
|
|
|
|
|
|
|
|
|
|
|
|
|
|
|
|
|
|
|
|
|
|
|
|
|
|
|
|
|
|
|
|
|
|
|
|
|
|
|
|
|
|
|
|
|
|
|
|
|
|
|
|
|
|
|
|
|
|
|
|
|
|
|
|
|
|
|
|
|
|
|
|
|
|
|
|
|
|
|
|
|
|
|
|
|
|
|
|
|
|
|
|
|
|
|
|
|
|
|
|
|
|
|
|
|
|
|
CH3 |
|
|
|
|
|
|
|
|
|
|
|
|
|
|
|
|
|
|
|
|
|
|
|
|
|
|
|
|
|
|
|
|
|
|
|
|
|
|
|
|||||
isoprene)– |
PDI- |
|
|
|
|
|
|
|
|
|
|
|
|
|
|
|
|
|
|
|
|
|
|
|
|
|
|
|
|
|
|
|
|
|
|
|
|
|
|
|
|
|
|
|
|
|
|
|
|
|
|
|
|
|
|
|
|
|
|
|
|
|
|
|
|
|
|
|
|
|
|
|
|
||||||||||||||
|
|
|
|
|
|
|
|
|
|
|
|
|
|
|
|
|
|
|
|
|
|
|
|
|
|
|
|
|
|
|
|
|
CH3 |
|
|
|
|
|
|
|
|
|
|
|
|
|
|
|
|
|
|
|
|
|
|
|
|
|
|
|
|
|
|
|
|
|
|
||||||||||||||||||||
urethane–epoxide |
3B |
|
|
|
|
|
|
|
|
|
|
|
|
|
|
|
|
|
|
|
|
|
|
|
|
|
|
|
|
|
|
|
|
|
|
|
|
C |
|
|
|
|
|
O |
|
|
CH |
|
|
|
CH |
|
|
CH2 |
|
||||||||||||||||||||||||||||||||
|
|
|
|
|
|
|
|
|
|
|
|
|
|
|
|
|
|
|
|
|
|
|
|
|
|
|
|
|
|
|
|
|
|
|
|
|
|
NH |
|
|
|
|
|
|
|
|
|
|
|
|
|
|
|||||||||||||||||||||||||||||||||||
|
|
|
|
|
|
|
|
|
|
|
|
|
|
|
|
|
|
|
|
|
|
|
|
|
|
|
|
|
|
|
|
|
|
|
|
|
|
|
|
|
|
|
|
|
|
|
|
2 |
|
|
|
|
|||||||||||||||||||||||||||||||||||
(polydiene– |
|
|
|
|
|
|
|
|
|
|
|
|
|
|
|
|
|
|
|
|
|
|
|
|
|
|
|
|
|
|
|
|
|
|
|
|
|
|
|
|
|
|
|
|
|
|
|
|
|
|
|
|
|
|
|
|
|
|
|
|
|
|
|
|
|
|
|
|
|
|
|
|
|
|
|
|
|
|
O |
|
|
|
|
|
|
||
|
|
|
|
|
|
|
|
|
|
|
|
|
|
|
|
|
|
|
|
|
|
|
|
|
|
|
|
|
|
|
|
|
|
|
|
|
|
|
|
|
|
|
|
|
|
|
|
|
|
|
|
|
|
|
|
O |
|
|
|
|
|
|
|
|
|
|
|
|
|
|
|
|
|
|
|
|
|||||||||||
|
|
|
|
|
|
|
|
|
|
|
|
|
|
|
|
|
|
|
|
|
|
|
|
|
|
|
|
|
|
|
|
|
|
|
|
|
|
|
|
|
|
|
|
|
|
|
|
|
|
|
|
|
|
|
|
|
|
|
|
|
|
|
|
|
|
|
|
|
|
|
|
|
|
|
|
|
|
|
|
|
|||||||
epoxide–urethane |
( |
CH |
|
CH |
|
|
CH |
|
|
CH |
|
|
) |
|
|
|
CH |
|
CH |
|
|
O |
|
|
|
|
C |
|
NH |
|
|
|
|
|
|
|
|
|
|
|
|
|
|
|
|
|
|
|
|
|
|
|
|
|
|
|
|
|
|
|
|
|
|
|
|
|
|
|
|||||||||||||||||||
m |
2 |
|
|
|
|
|
|
|
|
|
|
|
|
|
|
|
|
|
|
|
|
|
|
|
|
|
|
|
|
|
|
|
|
|
|
|
|
|
|
|
|
|
|
|
|||||||||||||||||||||||||||||||||||||||||||
2 |
|
|
|
|
|
|
|
|
|
|
|
|
|
|
|
|
|
|
|
|
|
|
|
|
|
|
|
|
|
|
|
|
|
|
|
|
|
|
|
|
|
|
|||||||||||||||||||||||||||||||||||||||||||||
2 |
|
|
|
|
|
|
|
|
|
|
|
|
|
|
|
|
|
|
|
|
|
|
|
|
|
|
|
|
|
|
|
|
|
|
|
|
|
|
|
|
|
|
|
|
|
|
|
|
|
|
|||||||||||||||||||||||||||||||||||||
|
|
|
|
|
|
|
|
|
|
|
|
|
|
|
|
|
|
|
|
|
|
|
|
|
|
|
|
CH3 |
|
|
|
|
|
|
|
|
|
|
|
|
|
|
|
|
|
|
|
|
|
|
|
|
|
|
|
|
|
|
|
|
|
|
|
|
|
|
|
|
|
|
|
|
|
|
|||||||||||||
|
|
|
|
|
|
|
|
|
|
|
|
|
|
|
|
|
|
|
|
|
|
|
|
|
|
|
|
|
O |
|
|
|
|
|
|
|
|
|
|
|
|
|
|
|
|
|
|
|
|
|
|
|
|
|
|
|
|
|
|
|
|
|
|
|
|
|
|
|
|
|
|
|
|
||||||||||||||
|
|
|
|
|
|
|
|
|
|
|
|
|
|
|
|
|
|
|
|
|
|
|
|
|
|
|
|
|
|
|
|
|
|
|
|
|
|
|
|
|
|
|
|
|
|
|
|
|
|||||||||||||||||||||||||||||||||||||||
EET-1 epoxy res- |
|
|
|
|
|
|
|
|
|
|
|
|
|
CH2 |
|
|
|
|
|
|
O |
|
|
|
|
|
|
|
CH2 |
|
|
|
|
|
|
|
|
|
CH |
|
|
|
|
|
|
|
|
|
|
CH2 |
|
|
|
|
|
|
|||||||||||||||||||||||||||||||
|
|
|
|
|
|
|
|
|
|
|
|
|
|
|
|
|
|
|
|
|
|
|
|
|
|
|
|
|
|
|
|
|
|
|
|
|
|
|
|
|
|
|
|
|
|
|
|
||||||||||||||||||||||||||||||||||||||||
in. Triol |
|
|
|
|
|
|
|
|
|
|
|
|
|
|
|
|
|
|
|
|
|
|
|
|
|
|
|
|
|
|
|
|
|
|
|
|
|
|
|
|
|
O |
|
|
|
|
|
|
|
|
|
|
|
|
|
|
|
|
|
|
|
|
|
O |
|
|
|
|
|
|
|
|
|
|
|
|
|
|
|
|
|
|
|
||||
triglycidyl ether |
|
|
C2H5 |
|
|
|
C |
|
|
|
|
CH2 |
|
|
|
|
|
|
|
|
|
CH2 |
|
|
|
|
CH |
|
|
|
CH2 |
|
|
|
|
||||||||||||||||||||||||||||||||||||||||||||||||||||
|
|
|
|
|
|
|
|
|
|
|
|
|
|
|
|
|
|
|
|
|
|
|
|
|
|
|
|
|
|
|
|
|
|
|
|
|
|
|
|
|
|
|
|
|
|
|
|
|
|
|
|||||||||||||||||||||||||||||||||||||
|
|
|
|
|
|
|
|
|
|
|
|
|
|
|
|
|
|
|
|
|
|
|
|
|
|
|
|
|
|
|
|
|
|
|
|
|
|
|
|
|
|
|
|
|
|
|
|
|
|
|
|
|
|
|
|
|
|
|
|
|
|
|
|
|
|
|
|
|
|
|
|
|
O |
|
|
|
|
|
|
||||||||
|
|
|
|
|
|
|
|
|
|
|
|
|
|
|
|
|
|
|
|
|
|
|
|
|
|
|
|
|
|
|
|
|
|
|
|
|
|
|
|
|
|
|
|
|
|
|
|
|
|
|
|
|
|
|
|
|
|
|
|
|
|
|
|
|
|
|
|
|
|
|
|
|
|
|
|
|
|
|
|
||||||||
|
|
|
|
|
|
|
|
|
|
|
|
|
|
CH2 |
|
|
|
|
|
O |
|
|
CH2 |
|
|
|
|
|
|
|
CH |
|
|
|
|
|
|
|
|
CH2 |
|
|
|
|
|
|
|
|
|
|
|
||||||||||||||||||||||||||||||||||||
|
|
|
|
|
|
|
|
|
|
|
|
|
|
|
|
|
|
|
|
|
|
|
|
|
|
|
|
|
|
O |
|
|
|
|
|
|
|
|
|
|
|
|
|||||||||||||||||||||||||||||||||||||||||||||
|
|
|
|
|
|
|
|
|
|
|
|
|
|
|
|
|
|
|
|
|
|
|
|
|
|
|
|
|
|
|
|
|
|
|
|
|
|
|
|
|
|
|
|
|
|
|
|
|
|
|
|
|
|
|
|
|
|
|
|
|
|
|
|
|
|
|
|
|
|
|
|
|
|
|
|
|
|
|
|
|
|
|
157

|
|
|
|
|
|
|
|
|
|
|
|
m |
|
|
2 |
|
|
|
|
|
|
|
|
|
|
|
|
|
|
W 13 RT 1 1,25 |
|
|
|
|
|
|
|
|
|
|
|
||||||||
|
|
|
|
|
|
|
|
|
|
|
|
|
|
|
|
|||||||||
|
|
|
|
|
|
ch r |
|
|
|
1 |
|
|
|
|
|
|
|
|
|
|
|
|||
|
|
|
|
|
|
|
|
|
|
|
|
|
|
|
|
|
|
|
|
|
|
|
||
|
|
|
|
|
|
|
|
|
|
|
|
m |
|
|
|
|
|
|
|
|
(1) |
|||
|
3 |
3 b 2 |
|
|
|
|
3 |
|
|
|
2 |
|
1 |
|
3 |
|
2 |
1 |
|
|||||
|
b |
|
|
|
T Tg |
|
|
|
2 b |
3 b |
|
|||||||||||||
|
|
|
|
|
29exp |
0,225 10 |
|
|
|
a |
|
|
|
|
|
|
|
|||||||
|
2 |
|
|
|
|
|
|
2 |
2 |
|
|
|||||||||||||
|
|
b |
|
|
|
|
|
|
|
|
|
|
|
|
|
|
b |
|
|
|
||||
|
|
|
|
|
|
|
|
|
|
|
|
|
|
|
|
|
|
|
|
|||||
|
|
Table 3. Optimum parameters of silicon dioxide fractions |
|
|
|
|||||||||||||||||||
|
|
|
|
|
|
|
Volume content |
|||||||||||||||||
Fraction no. |
|
Particle diameter, |
|
Volume fraction |
|
|
||||||||||||||||||
|
|
|
|
|
μm |
|
|
|
of pores |
|
|
|
|
0,079 |
of fraction |
|||||||||
|
1 |
|
|
|
1 |
|
|
0,465 |
|
|
|
|
|
|
|
|
|
|
|
|
||||
|
2 |
|
|
|
30 |
|
|
0,386 |
|
|
|
|
|
|
|
0,237 |
|
|
|
|
|
|||
|
3 |
|
|
|
600 |
|
0,360 |
|
|
|
|
|
|
|
0,684 |
|
|
|
|
|
af a0 (1 3 / m ) 3 / m ;
bf b0 (1 3 / m ) 3 / m ; |
(2) |
bf b0 (1 3 / m ),
where indices f and 0 refer to the filled and free states of the threedimensionally cross-linked polymeric binder.
Effective concentration of cross-links and failure energy. For using Eq. (1) in engineering for the development of new composites based on threedimensionally cross-linked elastomers, in particular, of waterproofi ng frost-resistant asphalt coating and material for deformation seams of automobile roads [8, 9], it is necessary to know the ultimate relative elongation or
breaking strain of the polymeric binder. As follows from (1), the quantities b0
and b0 are determined by the volume fraction of the polymer in the plasticized
binder φr and by the effective concentration of cross-links νeff, including permanent chemical cross-links (νch) and variable intermolecular (“physical”) cross-links (νph), with the “physical” cross-links determining the temperature dependence of the mechanical characteristics:
158

eff ch r1/3 1 ph (T Tg ) ch r1/3 1 29exp 0,225 10 3 (T Tg )2 , (3)
where Tg is the structural glass transition point of the polymeric binder, depending on the corresponding points of its components, including the plasticizer (taking into account its fraction), and also on the chemical structure of the polymer base network obtained. Equation (3) refl ects in the explicit form the viscoelastic behavior of polymeric binders and contains the most important physicochemical characteristics determining the dependence σ(α), namely, the concentration of chemical cross-links, the temperature dependence of the concentration of intermolecular cross-links, and the structural glass transition point of the polymeric binder. The parameters of the 3D molecular network obtainednfrom low-molecular-mass rubbers with terminal functional groups, including the statistical mean molecular mass of the chain segment between
cross-links Мs , were estimated theoretically in [10]. However, in so doing,
the intermolecular interaction was not taken into account. Like the mechanical characteristics, the intermolecular interaction, as already noted, depends on numerous factors [10–12]. Therefore, to determine the breaking strain of the free polymeric binder in relation to νeff, we used the experimental data obtained previously [1].
The mechanical failure energy was calculated for different concentrations of chemical bonds ensuring the maximal level of limiting strain characteristics. As example of practical application of Eq. (1), we used the chosen low-molecular-mass rubbers. The polymeric binder contained tributyl phosphate [PO(С4H9O)3] as plasticizer; its glass transition point is 193 K. The fi ller was silicon dioxide (quartz sand) in the form of a mixture of two, three, or four fractions. The optimum parameters of the fractions are given in Table 3. The relative strain velocity of 1.4 × 10–3 s–1 was chosen as standard. Theinitial data for calculating the mechanical failure energy are given in Table 4.
Figure 1 shows the dependence of the mechanical failure energy of the fi lled elastomer on the tensile strain of the specimen εb at different concentrations of chemical cross-links and different testing temperatures.
As can be seen, in contrast to the failure point envelopes according to Smith [5], the mechanical failure energy [7] refl ects more completely, from the physical viewpoint, the mechanical resistance of the filled elastomer, which is important for evaluation of its suitability for operation in specifi c materials or structures.
159
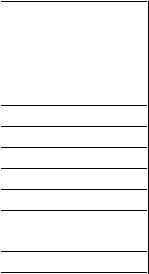
Table 4. Initial data for calculating the mechanical failure energy
Parameter |
Value |
Number of fractions |
4 |
Testing temperature, K |
223, 293, 323 |
Glass transition point of polymer, K |
194 |
Volume expansion coeffi cient of polymer |
5·10-4 |
|
7·10-4 |
Volume expansion coeffi cient of plasticizer |
|
Volume fraction of polymer in binder |
0,7; 0,5; 0,3 |
Glass transition point of polymeric binder, K |
193 |
Glass transition point of PCM, K |
193,65 |
Volume fraction of fi ller in composite |
0,65 |
Concentration of chemical cross-links in polymer base |
(0,1; 0,3; 0,5; 1,0; |
of the binder |
3,0; 5,0)·10-5 |
Maximal volume fi lling (calculated) |
0,84; 0,94; 0,96 |
Figure 1 also shows that, at 223 K, as the concentration of chemical crosslinks is increased from 0.1 × 10–5 to 1.0 × 10–5 mol cm3, the energy of the mechanical failure of the elastomer fi lled with polyfractional silicon dioxide increases from 900 to 1400 J, and the breaking strain decreases from 90 to 40%, respectively. At the testing temperature increased to 293 and 323 K, as the concentration of chemical cross-links is increased, the mechanical failure energy increases from 80 to 1400 J, and the breaking strain regularly decreases.
The tests show that, at low temperatures (of the order of 223 K), high level of the mechanical failure energy of the elastomer fi lled with polyfractional solid particles of silicon dioxide is ensured at chemical crosslinkconcentrations in the range (0.1–1.0) × 10–5 mol/ cm–3, and at thicker cross-linking the failure energy decreases to 900 and 600 J. At higher temperatures (293 to 323 K), an increase in the concentration of chemical cross-links to (3.0–5.0) × 10–5 mol/ cm–3 leads to an increase in the mechanical failure energy from 1300 to 1400 J.
Taking into account the results obtained, it is appropriate to use for building automobile roads indented for operation at temperatures in the interval 223– 323 K the elastomer fi lled with polyfractional silicon dioxide at the concentration of chemical cross-links in the interval (0.1–1.0) × 10–5 mol/ cm–3.
160