
- •Preface
- •Imaging Microscopic Features
- •Measuring the Crystal Structure
- •References
- •Contents
- •1.4 Simulating the Effects of Elastic Scattering: Monte Carlo Calculations
- •What Are the Main Features of the Beam Electron Interaction Volume?
- •How Does the Interaction Volume Change with Composition?
- •How Does the Interaction Volume Change with Incident Beam Energy?
- •How Does the Interaction Volume Change with Specimen Tilt?
- •1.5 A Range Equation To Estimate the Size of the Interaction Volume
- •References
- •2: Backscattered Electrons
- •2.1 Origin
- •2.2.1 BSE Response to Specimen Composition (η vs. Atomic Number, Z)
- •SEM Image Contrast with BSE: “Atomic Number Contrast”
- •SEM Image Contrast: “BSE Topographic Contrast—Number Effects”
- •2.2.3 Angular Distribution of Backscattering
- •Beam Incident at an Acute Angle to the Specimen Surface (Specimen Tilt > 0°)
- •SEM Image Contrast: “BSE Topographic Contrast—Trajectory Effects”
- •2.2.4 Spatial Distribution of Backscattering
- •Depth Distribution of Backscattering
- •Radial Distribution of Backscattered Electrons
- •2.3 Summary
- •References
- •3: Secondary Electrons
- •3.1 Origin
- •3.2 Energy Distribution
- •3.3 Escape Depth of Secondary Electrons
- •3.8 Spatial Characteristics of Secondary Electrons
- •References
- •4: X-Rays
- •4.1 Overview
- •4.2 Characteristic X-Rays
- •4.2.1 Origin
- •4.2.2 Fluorescence Yield
- •4.2.3 X-Ray Families
- •4.2.4 X-Ray Nomenclature
- •4.2.6 Characteristic X-Ray Intensity
- •Isolated Atoms
- •X-Ray Production in Thin Foils
- •X-Ray Intensity Emitted from Thick, Solid Specimens
- •4.3 X-Ray Continuum (bremsstrahlung)
- •4.3.1 X-Ray Continuum Intensity
- •4.3.3 Range of X-ray Production
- •4.4 X-Ray Absorption
- •4.5 X-Ray Fluorescence
- •References
- •5.1 Electron Beam Parameters
- •5.2 Electron Optical Parameters
- •5.2.1 Beam Energy
- •Landing Energy
- •5.2.2 Beam Diameter
- •5.2.3 Beam Current
- •5.2.4 Beam Current Density
- •5.2.5 Beam Convergence Angle, α
- •5.2.6 Beam Solid Angle
- •5.2.7 Electron Optical Brightness, β
- •Brightness Equation
- •5.2.8 Focus
- •Astigmatism
- •5.3 SEM Imaging Modes
- •5.3.1 High Depth-of-Field Mode
- •5.3.2 High-Current Mode
- •5.3.3 Resolution Mode
- •5.3.4 Low-Voltage Mode
- •5.4 Electron Detectors
- •5.4.1 Important Properties of BSE and SE for Detector Design and Operation
- •Abundance
- •Angular Distribution
- •Kinetic Energy Response
- •5.4.2 Detector Characteristics
- •Angular Measures for Electron Detectors
- •Elevation (Take-Off) Angle, ψ, and Azimuthal Angle, ζ
- •Solid Angle, Ω
- •Energy Response
- •Bandwidth
- •5.4.3 Common Types of Electron Detectors
- •Backscattered Electrons
- •Passive Detectors
- •Scintillation Detectors
- •Semiconductor BSE Detectors
- •5.4.4 Secondary Electron Detectors
- •Everhart–Thornley Detector
- •Through-the-Lens (TTL) Electron Detectors
- •TTL SE Detector
- •TTL BSE Detector
- •Measuring the DQE: BSE Semiconductor Detector
- •References
- •6: Image Formation
- •6.1 Image Construction by Scanning Action
- •6.2 Magnification
- •6.3 Making Dimensional Measurements With the SEM: How Big Is That Feature?
- •Using a Calibrated Structure in ImageJ-Fiji
- •6.4 Image Defects
- •6.4.1 Projection Distortion (Foreshortening)
- •6.4.2 Image Defocusing (Blurring)
- •6.5 Making Measurements on Surfaces With Arbitrary Topography: Stereomicroscopy
- •6.5.1 Qualitative Stereomicroscopy
- •Fixed beam, Specimen Position Altered
- •Fixed Specimen, Beam Incidence Angle Changed
- •6.5.2 Quantitative Stereomicroscopy
- •Measuring a Simple Vertical Displacement
- •References
- •7: SEM Image Interpretation
- •7.1 Information in SEM Images
- •7.2.2 Calculating Atomic Number Contrast
- •Establishing a Robust Light-Optical Analogy
- •Getting It Wrong: Breaking the Light-Optical Analogy of the Everhart–Thornley (Positive Bias) Detector
- •Deconstructing the SEM/E–T Image of Topography
- •SUM Mode (A + B)
- •DIFFERENCE Mode (A−B)
- •References
- •References
- •9: Image Defects
- •9.1 Charging
- •9.1.1 What Is Specimen Charging?
- •9.1.3 Techniques to Control Charging Artifacts (High Vacuum Instruments)
- •Observing Uncoated Specimens
- •Coating an Insulating Specimen for Charge Dissipation
- •Choosing the Coating for Imaging Morphology
- •9.2 Radiation Damage
- •9.3 Contamination
- •References
- •10: High Resolution Imaging
- •10.2 Instrumentation Considerations
- •10.4.1 SE Range Effects Produce Bright Edges (Isolated Edges)
- •10.4.4 Too Much of a Good Thing: The Bright Edge Effect Hinders Locating the True Position of an Edge for Critical Dimension Metrology
- •10.5.1 Beam Energy Strategies
- •Low Beam Energy Strategy
- •High Beam Energy Strategy
- •Making More SE1: Apply a Thin High-δ Metal Coating
- •Making Fewer BSEs, SE2, and SE3 by Eliminating Bulk Scattering From the Substrate
- •10.6 Factors That Hinder Achieving High Resolution
- •10.6.2 Pathological Specimen Behavior
- •Contamination
- •Instabilities
- •References
- •11: Low Beam Energy SEM
- •11.3 Selecting the Beam Energy to Control the Spatial Sampling of Imaging Signals
- •11.3.1 Low Beam Energy for High Lateral Resolution SEM
- •11.3.2 Low Beam Energy for High Depth Resolution SEM
- •11.3.3 Extremely Low Beam Energy Imaging
- •References
- •12.1.1 Stable Electron Source Operation
- •12.1.2 Maintaining Beam Integrity
- •12.1.4 Minimizing Contamination
- •12.3.1 Control of Specimen Charging
- •12.5 VPSEM Image Resolution
- •References
- •13: ImageJ and Fiji
- •13.1 The ImageJ Universe
- •13.2 Fiji
- •13.3 Plugins
- •13.4 Where to Learn More
- •References
- •14: SEM Imaging Checklist
- •14.1.1 Conducting or Semiconducting Specimens
- •14.1.2 Insulating Specimens
- •14.2 Electron Signals Available
- •14.2.1 Beam Electron Range
- •14.2.2 Backscattered Electrons
- •14.2.3 Secondary Electrons
- •14.3 Selecting the Electron Detector
- •14.3.2 Backscattered Electron Detectors
- •14.3.3 “Through-the-Lens” Detectors
- •14.4 Selecting the Beam Energy for SEM Imaging
- •14.4.4 High Resolution SEM Imaging
- •Strategy 1
- •Strategy 2
- •14.5 Selecting the Beam Current
- •14.5.1 High Resolution Imaging
- •14.5.2 Low Contrast Features Require High Beam Current and/or Long Frame Time to Establish Visibility
- •14.6 Image Presentation
- •14.6.1 “Live” Display Adjustments
- •14.6.2 Post-Collection Processing
- •14.7 Image Interpretation
- •14.7.1 Observer’s Point of View
- •14.7.3 Contrast Encoding
- •14.8.1 VPSEM Advantages
- •14.8.2 VPSEM Disadvantages
- •15: SEM Case Studies
- •15.1 Case Study: How High Is That Feature Relative to Another?
- •15.2 Revealing Shallow Surface Relief
- •16.1.2 Minor Artifacts: The Si-Escape Peak
- •16.1.3 Minor Artifacts: Coincidence Peaks
- •16.1.4 Minor Artifacts: Si Absorption Edge and Si Internal Fluorescence Peak
- •16.2 “Best Practices” for Electron-Excited EDS Operation
- •16.2.1 Operation of the EDS System
- •Choosing the EDS Time Constant (Resolution and Throughput)
- •Choosing the Solid Angle of the EDS
- •Selecting a Beam Current for an Acceptable Level of System Dead-Time
- •16.3.1 Detector Geometry
- •16.3.2 Process Time
- •16.3.3 Optimal Working Distance
- •16.3.4 Detector Orientation
- •16.3.5 Count Rate Linearity
- •16.3.6 Energy Calibration Linearity
- •16.3.7 Other Items
- •16.3.8 Setting Up a Quality Control Program
- •Using the QC Tools Within DTSA-II
- •Creating a QC Project
- •Linearity of Output Count Rate with Live-Time Dose
- •Resolution and Peak Position Stability with Count Rate
- •Solid Angle for Low X-ray Flux
- •Maximizing Throughput at Moderate Resolution
- •References
- •17: DTSA-II EDS Software
- •17.1 Getting Started With NIST DTSA-II
- •17.1.1 Motivation
- •17.1.2 Platform
- •17.1.3 Overview
- •17.1.4 Design
- •Simulation
- •Quantification
- •Experiment Design
- •Modeled Detectors (. Fig. 17.1)
- •Window Type (. Fig. 17.2)
- •The Optimal Working Distance (. Figs. 17.3 and 17.4)
- •Elevation Angle
- •Sample-to-Detector Distance
- •Detector Area
- •Crystal Thickness
- •Number of Channels, Energy Scale, and Zero Offset
- •Resolution at Mn Kα (Approximate)
- •Azimuthal Angle
- •Gold Layer, Aluminum Layer, Nickel Layer
- •Dead Layer
- •Zero Strobe Discriminator (. Figs. 17.7 and 17.8)
- •Material Editor Dialog (. Figs. 17.9, 17.10, 17.11, 17.12, 17.13, and 17.14)
- •17.2.1 Introduction
- •17.2.2 Monte Carlo Simulation
- •17.2.4 Optional Tables
- •References
- •18: Qualitative Elemental Analysis by Energy Dispersive X-Ray Spectrometry
- •18.1 Quality Assurance Issues for Qualitative Analysis: EDS Calibration
- •18.2 Principles of Qualitative EDS Analysis
- •Exciting Characteristic X-Rays
- •Fluorescence Yield
- •X-ray Absorption
- •Si Escape Peak
- •Coincidence Peaks
- •18.3 Performing Manual Qualitative Analysis
- •Beam Energy
- •Choosing the EDS Resolution (Detector Time Constant)
- •Obtaining Adequate Counts
- •18.4.1 Employ the Available Software Tools
- •18.4.3 Lower Photon Energy Region
- •18.4.5 Checking Your Work
- •18.5 A Worked Example of Manual Peak Identification
- •References
- •19.1 What Is a k-ratio?
- •19.3 Sets of k-ratios
- •19.5 The Analytical Total
- •19.6 Normalization
- •19.7.1 Oxygen by Assumed Stoichiometry
- •19.7.3 Element by Difference
- •19.8 Ways of Reporting Composition
- •19.8.1 Mass Fraction
- •19.8.2 Atomic Fraction
- •19.8.3 Stoichiometry
- •19.8.4 Oxide Fractions
- •Example Calculations
- •19.9 The Accuracy of Quantitative Electron-Excited X-ray Microanalysis
- •19.9.1 Standards-Based k-ratio Protocol
- •19.9.2 “Standardless Analysis”
- •19.10 Appendix
- •19.10.1 The Need for Matrix Corrections To Achieve Quantitative Analysis
- •19.10.2 The Physical Origin of Matrix Effects
- •19.10.3 ZAF Factors in Microanalysis
- •X-ray Generation With Depth, φ(ρz)
- •X-ray Absorption Effect, A
- •X-ray Fluorescence, F
- •References
- •20.2 Instrumentation Requirements
- •20.2.1 Choosing the EDS Parameters
- •EDS Spectrum Channel Energy Width and Spectrum Energy Span
- •EDS Time Constant (Resolution and Throughput)
- •EDS Calibration
- •EDS Solid Angle
- •20.2.2 Choosing the Beam Energy, E0
- •20.2.3 Measuring the Beam Current
- •20.2.4 Choosing the Beam Current
- •Optimizing Analysis Strategy
- •20.3.4 Ba-Ti Interference in BaTiSi3O9
- •20.4 The Need for an Iterative Qualitative and Quantitative Analysis Strategy
- •20.4.2 Analysis of a Stainless Steel
- •20.5 Is the Specimen Homogeneous?
- •20.6 Beam-Sensitive Specimens
- •20.6.1 Alkali Element Migration
- •20.6.2 Materials Subject to Mass Loss During Electron Bombardment—the Marshall-Hall Method
- •Thin Section Analysis
- •Bulk Biological and Organic Specimens
- •References
- •21: Trace Analysis by SEM/EDS
- •21.1 Limits of Detection for SEM/EDS Microanalysis
- •21.2.1 Estimating CDL from a Trace or Minor Constituent from Measuring a Known Standard
- •21.2.2 Estimating CDL After Determination of a Minor or Trace Constituent with Severe Peak Interference from a Major Constituent
- •21.3 Measurements of Trace Constituents by Electron-Excited Energy Dispersive X-ray Spectrometry
- •The Inevitable Physics of Remote Excitation Within the Specimen: Secondary Fluorescence Beyond the Electron Interaction Volume
- •Simulation of Long-Range Secondary X-ray Fluorescence
- •NIST DTSA II Simulation: Vertical Interface Between Two Regions of Different Composition in a Flat Bulk Target
- •NIST DTSA II Simulation: Cubic Particle Embedded in a Bulk Matrix
- •21.5 Summary
- •References
- •22.1.2 Low Beam Energy Analysis Range
- •22.2 Advantage of Low Beam Energy X-Ray Microanalysis
- •22.2.1 Improved Spatial Resolution
- •22.3 Challenges and Limitations of Low Beam Energy X-Ray Microanalysis
- •22.3.1 Reduced Access to Elements
- •22.3.3 At Low Beam Energy, Almost Everything Is Found To Be Layered
- •Analysis of Surface Contamination
- •References
- •23: Analysis of Specimens with Special Geometry: Irregular Bulk Objects and Particles
- •23.2.1 No Chemical Etching
- •23.3 Consequences of Attempting Analysis of Bulk Materials With Rough Surfaces
- •23.4.1 The Raw Analytical Total
- •23.4.2 The Shape of the EDS Spectrum
- •23.5 Best Practices for Analysis of Rough Bulk Samples
- •23.6 Particle Analysis
- •Particle Sample Preparation: Bulk Substrate
- •The Importance of Beam Placement
- •Overscanning
- •“Particle Mass Effect”
- •“Particle Absorption Effect”
- •The Analytical Total Reveals the Impact of Particle Effects
- •Does Overscanning Help?
- •23.6.6 Peak-to-Background (P/B) Method
- •Specimen Geometry Severely Affects the k-ratio, but Not the P/B
- •Using the P/B Correspondence
- •23.7 Summary
- •References
- •24: Compositional Mapping
- •24.2 X-Ray Spectrum Imaging
- •24.2.1 Utilizing XSI Datacubes
- •24.2.2 Derived Spectra
- •SUM Spectrum
- •MAXIMUM PIXEL Spectrum
- •24.3 Quantitative Compositional Mapping
- •24.4 Strategy for XSI Elemental Mapping Data Collection
- •24.4.1 Choosing the EDS Dead-Time
- •24.4.2 Choosing the Pixel Density
- •24.4.3 Choosing the Pixel Dwell Time
- •“Flash Mapping”
- •High Count Mapping
- •References
- •25.1 Gas Scattering Effects in the VPSEM
- •25.1.1 Why Doesn’t the EDS Collimator Exclude the Remote Skirt X-Rays?
- •25.2 What Can Be Done To Minimize gas Scattering in VPSEM?
- •25.2.2 Favorable Sample Characteristics
- •Particle Analysis
- •25.2.3 Unfavorable Sample Characteristics
- •References
- •26.1 Instrumentation
- •26.1.2 EDS Detector
- •26.1.3 Probe Current Measurement Device
- •Direct Measurement: Using a Faraday Cup and Picoammeter
- •A Faraday Cup
- •Electrically Isolated Stage
- •Indirect Measurement: Using a Calibration Spectrum
- •26.1.4 Conductive Coating
- •26.2 Sample Preparation
- •26.2.1 Standard Materials
- •26.2.2 Peak Reference Materials
- •26.3 Initial Set-Up
- •26.3.1 Calibrating the EDS Detector
- •Selecting a Pulse Process Time Constant
- •Energy Calibration
- •Quality Control
- •Sample Orientation
- •Detector Position
- •Probe Current
- •26.4 Collecting Data
- •26.4.1 Exploratory Spectrum
- •26.4.2 Experiment Optimization
- •26.4.3 Selecting Standards
- •26.4.4 Reference Spectra
- •26.4.5 Collecting Standards
- •26.4.6 Collecting Peak-Fitting References
- •26.5 Data Analysis
- •26.5.2 Quantification
- •26.6 Quality Check
- •Reference
- •27.2 Case Study: Aluminum Wire Failures in Residential Wiring
- •References
- •28: Cathodoluminescence
- •28.1 Origin
- •28.2 Measuring Cathodoluminescence
- •28.3 Applications of CL
- •28.3.1 Geology
- •Carbonado Diamond
- •Ancient Impact Zircons
- •28.3.2 Materials Science
- •Semiconductors
- •Lead-Acid Battery Plate Reactions
- •28.3.3 Organic Compounds
- •References
- •29.1.1 Single Crystals
- •29.1.2 Polycrystalline Materials
- •29.1.3 Conditions for Detecting Electron Channeling Contrast
- •Specimen Preparation
- •Instrument Conditions
- •29.2.1 Origin of EBSD Patterns
- •29.2.2 Cameras for EBSD Pattern Detection
- •29.2.3 EBSD Spatial Resolution
- •29.2.5 Steps in Typical EBSD Measurements
- •Sample Preparation for EBSD
- •Align Sample in the SEM
- •Check for EBSD Patterns
- •Adjust SEM and Select EBSD Map Parameters
- •Run the Automated Map
- •29.2.6 Display of the Acquired Data
- •29.2.7 Other Map Components
- •29.2.10 Application Example
- •Application of EBSD To Understand Meteorite Formation
- •29.2.11 Summary
- •Specimen Considerations
- •EBSD Detector
- •Selection of Candidate Crystallographic Phases
- •Microscope Operating Conditions and Pattern Optimization
- •Selection of EBSD Acquisition Parameters
- •Collect the Orientation Map
- •References
- •30.1 Introduction
- •30.2 Ion–Solid Interactions
- •30.3 Focused Ion Beam Systems
- •30.5 Preparation of Samples for SEM
- •30.5.1 Cross-Section Preparation
- •30.5.2 FIB Sample Preparation for 3D Techniques and Imaging
- •30.6 Summary
- •References
- •31: Ion Beam Microscopy
- •31.1 What Is So Useful About Ions?
- •31.2 Generating Ion Beams
- •31.3 Signal Generation in the HIM
- •31.5 Patterning with Ion Beams
- •31.7 Chemical Microanalysis with Ion Beams
- •References
- •Appendix
- •A Database of Electron–Solid Interactions
- •A Database of Electron–Solid Interactions
- •Introduction
- •Backscattered Electrons
- •Secondary Yields
- •Stopping Powers
- •X-ray Ionization Cross Sections
- •Conclusions
- •References
- •Index
- •Reference List
- •Index

20.6 · Beam-Sensitive Specimens |
|
|
|
|
|
|
|
|
331 |
|
20 |
||
|
|
|
|
|
|
|
|
|
|
|
|||
|
|
|
|
|
|
|
21.5-Mo_78.5-W |
|
|
||||
|
60 000 |
|
|
|
|
|
|
|
|
|
|
||
|
|
|
|
|
|
|
|
|
21.5-Mo_78.5-W_residual |
||||
Counts |
40 000 |
|
|
|
|
|
|
|
|
|
|
|
|
|
|
|
|
|
|
|
|
|
|
|
|
|
|
|
20 000 |
|
|
|
|
|
|
|
|
|
|
|
|
|
00.0 |
1.0 |
2.0 |
3.0 |
4.0 |
5.0 |
6.0 |
7.0 |
8.0 |
9.0 |
10.0 |
||
|
|
|
|
|
|
Photon Energy (keV) |
|
|
|
|
|
|
. Fig. 20.13 EDS spectrum of 0.215-Mo, 0.785-W alloy showing the residuals after peak fitting; E0 = 20 keV
. Table 20.12 Analysis of 0.215-Mo_0.785-W alloy (normalized mass fractions) (analysis in bold used for the calculations above)
Mo |
W |
|
|
0.2166 |
0.7834 |
|
|
0.2148 |
0.7852 |
0.2141 |
0.7859 |
0.2177 |
0.7823 |
0.2185 |
0.7815 |
0.2180 |
0.7820 |
0.2175 |
0.7825 |
0.2203 |
0.7797 |
0.2275 |
0.7725 |
0.2312 |
0.7688 |
0.2346 |
0.7654 |
0.2363 |
0.7637 |
0.2358 |
0.7642 |
0.2344 |
0.7656 |
0.2389 |
0.7611 |
Mo C = 0.2148 ± 0.0006 or 0.215 ± 0.28 % W C = 0.7852 ± 0.0024 or 0.785 ± 0.31 %
If multiple locations are measured under consistent measurement conditions—e.g., constant beam energy, beam current, and EDS performance—then values that fall outside the ranges given for Mo and W are indicative of heterogeneity, that is, real deviations in the composition of the alloy.
. Table 20.12 lists 15 measurements on this alloy made at randomly selected locations, which reveal significant heterogeneity with the most extreme excursion approximately 11% in the
Mo constituent from the ideal values. This deviation is well outside that expected from natural variations due to statistical fluctuations in the measured counts as calculated above.
The full uncertainty budget reported by DTSA-II, including the estimates for the uncertainties in the A and Z matrix corrections as well as the X-ray statistics is
Mo W σC = 0.0039 σC = 0.0017
The large increase in σC for Mo beyond the contribution of the X-ray statistics is due to the contribution of the matrix correction factor for absorption, A = 0.528.
20.6\ Beam-Sensitive Specimens
In some cases, the interaction of the electron beam can damage the specimen and locally alter the composition, often with the effects showing a strong dependence on the total dose, the dose per unit volume, and the dose rate.
20.6.1\ Alkali Element Migration
In some insulating materials, especially non-crystalline materials such as glasses, alkali family elements can migrate in response to the local charge injected below the surface by the beam, even when a thin conducting surface layer such as carbon has been applied to discharge the specimen. Migration typically leads to diminishing alkali concentration with time in the excited volume. The phenomenon can be detected by measuring a time series of spectra and carefully comparing the intensity of alkali element peaks to stable matrix peaks such as that of Si, as shown in . Fig. 20.14 for “Corning glass A” which has a high alkali composition with approximately 10 weight percent Na and 2.4 weight percent potassium (listed in
. Table 20.11) (Vicenzi et al. 2002). Each spectrum shown in
. Fig. 20.14 was recorded for 10 s with a fixed, focused beam, which creates the maximum possible dose per unit volume.
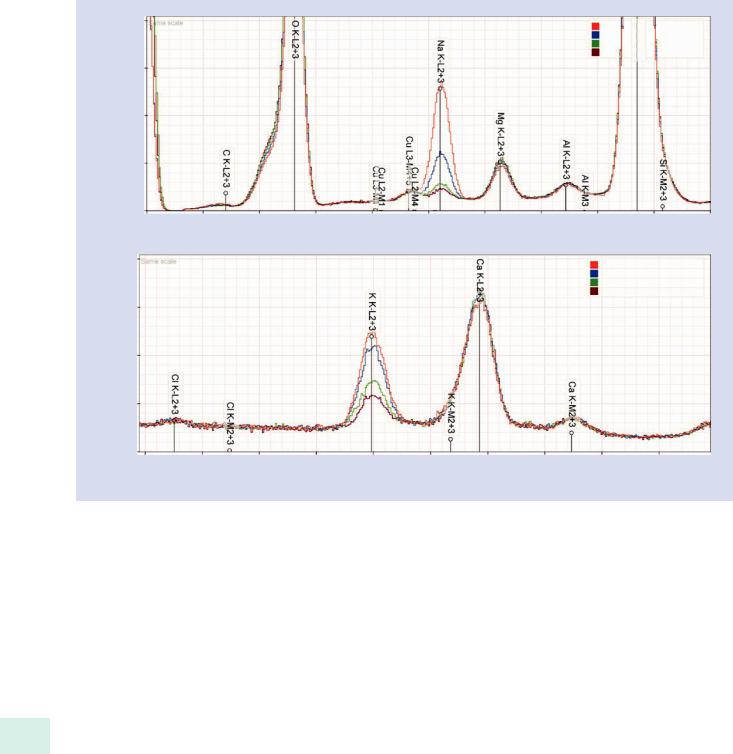
\332 Chapter 20 · Quantitative Analysis: The SEM/EDS Elemental Microanalysis k-ratio Procedure for Bulk Specimens, Step-by-Step
|
8 000 |
|
6 000 |
Counts |
4 000 |
|
|
|
2 000 |
|
0 |
|
0.0 |
|
2 000 |
|
1 500 |
Counts |
1 000 |
|
|
|
500 |
0
2.5
Corning Glass A E0 = 15 keV Fixed beam 150 nA-s
300 nA-s
750 nA-s
1500 nA-s
CorningA ka nt_10s_15kV15nA
_poi Si CorningA_point_20s_15kV15nA
CorningA_poi1+2 nt_50s_15kV15nA CorningA_point_100s_15kV15nA
0.2 |
0.4 |
0.6 |
0.8 |
1.0 |
1.2 |
1.4 |
1.6 |
1.8 |
2.0 |
|
|
|
|
Photon energy (keV) |
|
|
|
|
|
|
|
|
|
|
|
|
CorningA_point_10s_15kV15nA |
||
|
Corning Glass A |
|
|
|
|
CorningA_point_20s_15kV15nA |
|||
|
|
|
|
|
|
|
CorningA_point_50s_15kV15nA |
E0 = 15 keV |
CorningA_point_100s_15kV15nA |
|
|
Fixed beam |
|
150 nA-s |
|
300 nA-s |
|
750 nA-s |
|
1500 nA-s |
|
2.7 |
2.9 |
3.1 |
3.3 |
3.5 |
3.7 |
3.9 |
4.1 |
4.3 |
Photon energy (keV)
. Fig. 20.14 Corning glass A, showing Na and K migration as a function of dose for a fixed beam (15 keV, 15 nA)
Comparing the first (150-nA-s dose) and the second spectra (300-nA-s dose), the Na intensity is seen to fall by more than a factor of two as the dose increases, while the K intensity diminishes by approximately 20%. After a dose of 1500 nA-s, the Na peak is reduced to approximately 10% of its intensity after 10 s, while the K peak decreases to approximately 25 % of its original value, whereas other non-alkali elements—e.g., Mg, Al, Ca, Si, etc.—remain nearly constant with dose. Even this time series is somewhat misleading. If the initial dose is reduced by a factor of 10, the Na intensity observed is higher by approximately 30%, as shown in . Fig. 20.15, while the K
20 intensity is higher by approximately 5%. At the extremely high volumetric dose created by the fixed point beam in these experiments, significant alkali migration occurs even with the initial short beam dwell (e.g., 1 s, 15 nA). The effects of the dose on the results obtained by quantitative analysis with DTSA-II are given in . Table 20.13. Even in the first analysis (150 nA-s dose), the measured Na concentration is a factor of 2 lower than the synthesized glass composition, and after the maximum dose utilized for this series (1500 nA-s dose), the
Na concentration has decreased by a factor of 11.
Methods to reduce alkali element migration are based on modifying the total dose, the dose per unit area (and volume), and/or the dose rate. Reducing the dose per unit area is often one of the most effective ways to control migration. By defocusing the fixed beam or by scanning the focused beam rapidly over a large area, the dose per unit area can be greatly reduced, often by several orders of magnitude, compared to a fixed, focused beam. Because of the basic assumption of the k-ratio/matrix correction protocol that the material being analyzed must have the same composition over the entire volume excited by the electron beam, this increased-area strategy is only valid providing the region of analytical interest is homogeneous over a sufficiently large to accommodate the defocused or rapidly scanned beam. The effect of increasing the scanned area is shown in . Fig. 20.16 for Corning glass A, where the measured Na intensity increases rapidly as the scanned area is increased. . Table 20.14 compares DTSA-II quantitative analyses of spectra with the same dose (15 keV, 1500 nA-s) obtained with a point beam and with that beam rapidly scanning over an area 100 μm square. The scanned area results correspond very closely to the as-synthesized

20.6 · Beam-Sensitive Specimens
Counts
333 |
|
20 |
|
|
|
CorningA_point_10s_15kV15nA
CorningA_point_1s_15kV15nA
Corning Glass A E0 = 15 keV
15 nA-s
150 nA-s
0.0 |
0.2 |
0.4 |
0.6 |
0.8 |
1.0 |
1.2 |
1.4 |
1.6 |
1.8 |
2.0 |
|
|
|
|
|
Photon energy (keV) |
|
|
|
|
Counts
CorningA_point_10s_15kV15nA
CorningA_point_1s_15kV15nA
Corning Glass A E0 = 15 keV
15 nA-s
150 nA-s
2.5 |
2.7 |
2.9 |
3.1 |
3.3 |
3.5 |
3.7 |
3.9 |
4.1 |
4.3 |
Photon energy (keV)
. Fig. 20.15 Corning glass A, showing Na and K migration compared as a function of dose with a reduction of a factor of 10 difference for a fixed beam (15 keV, 15 nA); spectra normalized to the Si peak
. Table 20.13 DTSA-II analysis of Corning Glass A (E0 = 15 keV), oxygen by assumed stoichiometry, fixed beam
Element |
As-synthesized |
150 nA-s raw mass |
300 nA-s raw mass |
750 nA-s raw mass |
1500 nA-s raw mass |
|
mass conc |
conc |
conc |
conc |
conc |
|
|
|
|
|
|
O |
0.4421 |
0.4316 ± 0.0009 |
0.4313 ± 0.0009 |
0.4496 ± 0.0009 |
0.4644 ± 0.0009 |
|
|
|
|
|
|
Na |
0.1061 |
0.0519 ± 0.0004 |
0.0364 ± 0.0004 |
0.0172 ± 0.0003 |
0.0098 ± 0.0003 |
Mg |
0.0160 |
0.0161 ± 0.0002 |
0.0161 ± 0.0002 |
0.0178 ± 0.0002 |
0.0186 ± 0.0002 |
Al |
0.0529 |
0.0050 ± 0.0001 |
0.0051 ± 0.0001 |
0.0052 ± 0.0001 |
0.0058 ± 0.0001 |
Si |
0.3111 |
0.3192 ± 0.0006 |
0.3230 ± 0.0007 |
0.3438 ± 0.0007 |
0.3574 ± 0.0007 |
K |
0.0238 |
0.0251 ± 0.0003 |
0.0230 ± 0.0003 |
0.0195 ± 0.0003 |
0.0166 ± 0.0003 |
Ca |
0.0359 |
0.0363 ± 0.0003 |
0.0364 ± 0.0003 |
0.0381 ± 0.0003 |
0.0386 ± 0.0003 |
Ti |
0.00474 |
0.0053 ± 0.0002 |
0.0059 ± 0.0002 |
0.0057 ± 0.0002 |
0.0057 ± 0.0002 |
Mn |
0.00775 |
0.0086 ± 0.0003 |
0.0084 ± 0.0003 |
0.0091 ± 0.0003 |
0.0101 ± 0.0003 |
Fe |
0.00762 |
0.0083 ± 0.0003 |
0.0082 ± 0.0003 |
0.0074 ± 0.0003 |
0.0090 ± 0.0003 |
Cu |
0.00935 |
0.0098 ± 0.0005 |
0.0104 ± 0.0005 |
0.0112 ± 0.0005 |
0.0108 ± 0.0005 |
Sn |
0.00150 |
0.0030 ± 0.0008 |
0.0034 ± 0.0007 |
0.0036 ± 0.0007 |
0.0054 ± 0.0007 |
Sb |
0.0146 |
0.0124 ± 0.0007 |
0.0140 ± 0.0007 |
0.0139 ± 0.0007 |
0.0140 ± 0.0007 |
Ba |
0.0050 |
0.0045 ± 0.0005 |
0.0036 ± 0.0005 |
0.0056 ± 0.0005 |
0.0042 ± 0.0005 |
Raw total |
|
0.938 |
0.926 |
0.9485 |
0.9718 |